New/updated @ eXtension
Undercover Nutrient Investigation: The Effects of Mulch on Nutrients for Blueberry Webinar
Watch the webinar on YouTube at https://www.youtube.com/watch?v=A9l5qhyg-04
About the webinar
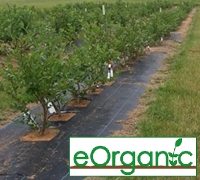
Learn about ongoing research from Oregon State University's Organic Blueberry Project. Research team members Dr. Dan Sullivan, Ryan Costello, and Luis Valenzuela of Oregon State University will discuss nutrient monitoring techniques used to measure mulch effects on nutrient availability, salts, and pH. Small scale methods for compost recipe development for blueberry will also be highlighted.
About eOrganic
eOrganic is the Organic Agriculture Community of Practice at eXtension.org. Our website at http:www.extension.org/organic_production contains articles, videos, and webinars for farmers, ranchers, agricultural professionals, certifiers, researchers and educators seeking reliable information on organic agriculture, published research results, farmer experiences, and certification. The content is collaboratively authored and reviewed by our community of University researchers and Extension personnel, agricultural professionals, farmers, and certifiers with experience and expertise in organic agriculture.
This is an eOrganic article and was reviewed for compliance with National Organic Program regulations by members of the eOrganic community. Always check with your organic certification agency before adopting new practices or using new materials. For more information, refer to eOrganic's articles on organic certification.
eOrganic 4935
ABCs of Organic Certification Webinar
Watch the video on YouTube at https://www.youtube.com/watch?v=ZiYAsSDrgB4
About the webinar
Thinking about getting certified, but don't know where to start? Have questions about the USDA organic standards? Join national organic expert, Jim Riddle, University of Minnesota, as he explains the National Organic Program crop production requirements and certification process. Good for growers, Extension agents, ag professionals, and organic food eaters.
About Jim Riddle
Jim Riddle has worked for over 26 years as an organic farmer, inspector, author, policy analyst and educator. He was founding chair of the International Organic Inspectors Association, (IOIA), and co-author of the IFOAM/IOIA International Organic Inspection Manual. He has trained hundreds of organic inspectors throughout the world. Jim served on the Minnesota Department of Agriculture’s Organic Advisory Task Force from 1991-2009, and was instrumental in passage of Minnesota’s landmark organic certification cost-share program. Since January 2006, Jim has worked as the University of Minnesota’s Organic Outreach Coordinator. Jim is former chair of the USDA’s National Organic Standards Board, and is a leading voice for organic agriculture.
About eOrganic
eOrganic is the Organic Agriculture Community of Practice at eXtension.org. Our website at http:www.extension.org/organic_production contains articles, videos, and webinars for farmers, ranchers, agricultural professionals, certifiers, researchers and educators seeking reliable information on organic agriculture, published research results, farmer experiences, and certification. The content is collaboratively authored and reviewed by our community of University researchers and Extension personnel, agricultural professionals, farmers, and certifiers with experience and expertise in organic agriculture.
This is an eOrganic article and was reviewed for compliance with National Organic Program regulations by members of the eOrganic community. Always check with your organic certification agency before adopting new practices or using new materials. For more information, refer to eOrganic's articles on organic certification.
eOrganic 4876
Organic Farming Financial Benchmarks Webinar by eOrganic
Watch the video on YouTube at https://www.youtube.com/watch?v=CC7h1nA-fUY
Resources from the Webinar
- FINBIN: http://www.finbin.umn.edu/
- Center for Farm Financial Management: http://www.cffm.umn.edu/
About the webinar
While there is much anecdotal information about farm performance and profitability, real world information about production, financial performance, and profitability on organic farms is scarce.
Since 2006, researchers at the University of Minnesota’s Center for Farm Financial Management and their partners have been collecting and analyzing farm financial data on organic farms and integrating them into FINBIN, one of the largest and most accessible sources of farm financial and production benchmark information in the world. Through their work, they are documenting the financial performance of participating organic producers so that they and their peers have more accurate benchmarks on which to evaluate organic options, as well as to un-mask the financial performance of a group of organic producers to provide agricultural lenders better information on which to base credit decisions and to give policy makers, researchers, and others real-world insight into organic farm performance.
In this webinar, Dale Nordquist, Center for Farm Financial Management at the University of Minnesota, will describe the Minnesota Organic Farm Business Management Project, and show three years worth of results of organic farm financial performance in Minnesota.
About eOrganic
eOrganic is the Organic Agriculture Community of Practice at eXtension.org. Our website at http:www.extension.org/organic_production contains articles, videos, and webinars for farmers, ranchers, agricultural professionals, certifiers, researchers and educators seeking reliable information on organic agriculture, published research results, farmer experiences, and certification. The content is collaboratively authored and reviewed by our community of University researchers and Extension personnel, agricultural professionals, farmers, and certifiers with experience and expertise in organic agriculture.
This is an eOrganic article and was reviewed for compliance with National Organic Program regulations by members of the eOrganic community. Always check with your organic certification agency before adopting new practices or using new materials. For more information, refer to eOrganic's articles on organic certification.
eOrganic 4855
Organic Late Blight Management 2009 Webinar by eOrganic
Watch the video on YouTube at https://www.youtube.com/watch?v=21-3WwRYiME
Late blight is a serious disease of potato and tomato family (Solanaceous) crops worldwide that can be difficult to control organically. eOrganic presenters Dr. Sally Miller of Ohio State University, Dr. Meg McGrath of Cornell University, and Dr. Alex Stone of Oregon State University discussed the 2009 epidemic and how to diagnose, prevent, and manage late blight on organic farms.
For additional webinars on late blight presented in 2010, see:
Late Blight Management on Organic Farms: 2010 Webinar
Late Blight Control in Your Organic Garden: 2010
This is an eOrganic article and was reviewed for compliance with National Organic Program regulations by members of the eOrganic community. Always check with your organic certification agency before adopting new practices or using new materials. For more information, refer to eOrganic's articles on organic certification.
eOrganic 4868
Pollination and Fertilization in Organic Seed Production
eOrganic authors:
John Navazio, Organic Seed Alliance and Washington State University
Frank Morton, Wild Garden Seed
Micaela Colley, Organic Seed Alliance
Linda Brewer, Oregon State University
Alex Stone, Oregon State University
This is an Organic Seed Resource Guide article.
Understanding the Reproductive Cycle of Seed CropsThere are several key reproductive steps in the life cycle of a flowering plant that results in adequate seed set. Pollination and subsequent fertilization of the ovules in the fruit are crucial processes in producing viable seed. There are a number of environmental challenges that can disrupt these processes and result in poor quality and quantity of a seed crop. Learning the basic steps of the reproductive process is very important in learning how to improve both the quality and quantity of the seed that you produce.
Squash flower pollination. Photo credit: Micaela Colley, Organic Seed Alliance.
Pollination, the movement of pollen from the anthers to the stigma, is essential for seed set and therefore crucial in seed production. An important consideration for any seed grower to know is whether the seed crop species that they are producing is predominately self-pollinated or cross-pollinated. Self–pollinated species have evolved to have perfect flowers that remain closed throughout the pollination process. These perfect flowers (bearing both male and female sexual parts on each flower) have anthers which are borne close to the stigma, allowing easy transfer of pollen to the stigmatic surface. This short journey for pollen to move from anther to stigma of the same flower (thus self-pollinating) often requires some external movement like wind to stimulate good pollen coverage of the stigma. In some selfers (runner beans and favas are good examples) insect visitation, even when the insect is unable to open the flower, can substantially increase seed set through their movement. Any grower producing a tomato crop in the greenhouse knows that the plants need a physical shaking or stiff air flow in order to achieve optimum pollination and subsequent fruit set on their crop.
Cross-pollinated species require genetic mixing between individuals of a population in order to remain genetically sound. All cross-pollinated crop species rely on either wind or insects (and occasionally animals) for pollen movement between individuals. All cross-pollinated crop species have flowers that open before pollen shed and receptivity. It is crucial that all of these species have adequate pollen availability during the flowering period. This requires; 1) a large enough population of the crop flowering in unison, 2) adequate insect populations present and visiting the crop or wind/airflow that is sufficient to move enough pollen for optimum pollination, and 3) environmental conditions that are such that pollen remains viable from the time of pollen shed until it reaches the flowers of other individuals in the population.
Fertilization and Seed FormationThe next step in this process leads to the fertilization of the ovules which become the seeds. After the pollen lands on the stigma, the receptive tip of the female parts of the flower, it must germinate and form a pollen tube which grows down through the style to reach the ovary. Each pollen tube that successfully reaches the ovary delivers one male gamete to fertilize an egg cell and one to fertilize the polar nuclei in a single ovule resulting in the formation of one seed. This fertilization event requires favorable environmental conditions and for fruit with multiple ovules a number of independent fertilization events must occur to insure good seed set. Once the embryo and endosperm form as a result of fertilization the seed undergoes a period of rapid cell division and growth. In most seed crops this growth and maturation of seed occurs in 40 to 60 days.
Problems in PollinationUpon release of the pollen from the anthers, the environmental conditions must be such that the pollen grain remains viable until it reaches the stigma of a flower of the same species. If it is too hot, the pollen may be denatured; if it is too dry, the pollen may desiccate and lose viability before reaching a receptive stigma. If it is too cool or rainy, the activity of pollinating insects can be reduced; honey bees are especially sensitive to these conditions and will not fly when it is too cool or wet. Rainy conditions can also impede the movement of pollen in wind-pollinated species as it can wet the pollen as anthers open and wash much of the pollen to the ground or make it immobile in wind pollinated species.
Another condition that can impede pollination for all self-pollinated species or wind-pollinated cross-pollinated species is to have little or no airflow at the time of pollen maturation and release. In self-pollinating plants, the anthers are always borne in close proximity to the stigma within the closed flowers common to all selfers. In some cases it is so close that just the act of dehiscence (the opening of the anther to release the pollen) will cause the pollen to fall onto the stigma with little or no prompting. However, in many cases this short journey requires some type of external movement to literally shake the pollen from the anthers onto the stigma. In many selfing species (tomatoes, peppers, common bean, peas) this is easily accomplished when the plant is grown outdoors by the movement of the plant in the wind. In some selfers (runner beans and favas are good examples) insect visitation, even when the insect is unable to open the flower, can substantially increase seed set through their movement. Any grower producing a tomato crop in the greenhouse knows that the plants need a physical shaking or stiff air flow in order to achieve optimum pollination and subsequent fruit set on their crop. When wind-pollinated crossers like corn, spinach, or beets are grown in the absence of normal wind and airflow during flowering, several days of unusually still air can hinder full seed set (or random mating across the population for the genetic mixing that is essential for crossers) due to low pollen flow in the air.
Problems in FertilizationFrom the time that the pollen comes in contact with the stigma, there are a number of problems that can arise. If the ambient temperatures are too high the pollen can become denatured and if it’s too low then the pollen will just sit until the temperature rises, although the flower’s receptivity is short lived. If the relative humidity is too low at this stage then the stigma or the pollen can desiccate, preventing the germination of the pollen. Low relative humidity has been found to be the culprit in a poor seed set for these reasons in a number of instances in vegetable seed production in the arid western states. The next step in the process of fertilization, the pollen tube growing down through the style can also be derailed by unfavorable weather conditions. The pollen tube is essentially a free living miniature plant (the gametophyte generation) and requires temperatures similar to the mother plant to grow vigorously. The pollen tube’s life cycle is usually 24 hours or less and it must make the trip from stigma to ovule in this period or not be successful in fertilizing the ovule. If the ambient temperature during this short period of time is colder or hotter than temperatures favorable to normal growth of the species than the pollen tube will stop growing and fail restart when the temperature comes back into a favorable range for growth. This will result in no fertilization for that particular pollen tube. In a cool loving crop like spinach that produces luxuriant growth at 58 – 65F (15 – 18C) and virtually stops growth at 78F (25.5C), this means that when it gets hotter than 78F (25.5C) as spinach seed crops are flowering there can be serious damage done to the yield due to poor fertilization of the ovules. Alternately, a heat loving crop like tomatoes can suffer blossom drop producing fewer fruit with low seed yields when tomatoes are exposed to cold night time temperatures during flowering.
Figure 1. Pollinating mechanisms and systems in common vegetable crops.
Crop Species
Primary Pollinating Mechanism(s)
Pollinating system
Wild Crossable Species in US
Self #1: self pollinating species, outcrossing is usually < 1%
Self #2: self-pollinating species that often outcross between 2-5%
Self #3: self-pollinating species that may cross at rates > 5%
Web Resources
- Bees and pollination [Online]. C. Britton. Ohio Agriculture Research and Development Center. The Ohio State University. Available at: http://osu.campusguides.com/agnic_bees_pollination (verified 11 June 2015).
- Evaluating honey bee colonies for pollination: a guide for growers and beekeepers [Online]. M. Burgett. 1984. Pacific Northwest Extension Publication 245. Available at: http://ir.library.oregonstate.edu/xmlui/handle/1957/16802 (verified 1 April 2011).
- Pollination [Online]. Honeybee Program and Entomology Department. University of Georgia. Available at: http://www.caes.uga.edu/departments/ent/bees/pollination/background.html (verified 1 April 2011).
- Insect pollination of cultivated crop plants [Online]. S.E. McGregor. Originally published in 1976. USDA Handbook 496. The first and only continuously updated virtual beekeeping book. Available at: https://www.ars.usda.gov/ARSUserFiles/20220500/OnlinePollinationHandbook.pdf (verified 14 June 2019).
- The Pollination Home Page [Online]. Pollination information and images. Includes state-by-state listings of beekeepers with bees available for contract pollination. Available at: http://pollinator.com/ (verified 1 April 2011).
- The Xerces Society Pollinator Conservation Program [Online]. Contains excellent downloadable or print information on how to provide habitat for native pollinators on your farm. Available at: http://www.xerces.org/pollinator-conservation/ (verified 1 April 2011).
- Farming for bees: guidelines for providing native bee habitat on farms [Online]. M. Vaughan, M. Shepherd, C. Kremen and S. H. Black. 2007. Available at: http://www.xerces.org/guidelines-farming-for-bees/ (verified 1 April 2011).
- Pacific Northwest plants for native bees [Online]. M. Shepherd. Undated. Available at: http://www.xerces.org/wp-content/uploads/2008/11/pnw_plants_bees_xerces_... (verified 1 April 2011).
- California plants for native bees [Online]. M. Shepherd. Undated. Available at: http://www.xerces.org/wp-content/uploads/2008/11/california_plants_for_n... (verified 1 April 2011).
- Using farm bill programs for pollinator conservation (draft) [Online]. M. Vaughn and M. Skinner. 2008. Available at: http://www.xerces.org/wp-content/uploads/2008/11/using_farm_bill_program... (verified 1 April 2011).
- Xerces Society fact sheets on native bee pollination of specific agricultural crops:
- Native bee pollination of watermelon [Online]. C. Kremen, N. Williams, S. Greenleaf, and R. Thorp. Undated. Available at: http://www.xerces.org/wp-content/uploads/2008/10/factsheet_watermelon_po... (verified 1 April 2011).
- Native bee pollination of cherry tomatoes [Online]. C. Kremen, N. Williams, S. Greenleaf, and R. Thorp. Undated. Available at: http://www.xerces.org/wp-content/uploads/2008/10/factsheet_cherry_tomato... (verified 1 April 2011).
- Native bee pollination of hybrid sunflowers [Online]. C. Kremen, N. Williams, S. Greenleaf, and R. Thorp. Undated. Available at: http://www.xerces.org/wp-content/uploads/2008/10/factsheet_sunflower_pol... (verified 1 April 2011).
- Crop pollination by bees. K.S. Delaplane and D.F. Mayer. 2000. CABI Press, New York, NY.
- Insect pollination of crops. J.B. Free. 1993. Academic Press, London, UK, and San Diego, CA.
- Pollinator conservation handbook. 2003. The Xerces Society. Portland, OR.
This is an eOrganic article and was reviewed for compliance with National Organic Program regulations by members of the eOrganic community. Always check with your organic certification agency before adopting new practices or using new materials. For more information, refer to eOrganic's articles on organic certification.
eOrganic 422
Organic Certification and Soil Conservation Compliance
eOrganic authors:
Michelle Wander, University of Illinois
Susan Andrews, USDA - Natural Resource Conservation Service
This article reviews the conservation goals for organic farming systems and considers how well organic certification standards line up with the Natural Resources Conservation Services's programs (EQIP, transition payments) and objectives. It provides research results from an Integrated Organic Program study evaluating organic transiton strategies and compares measured trends in soil organic matter with ranks produced by NRCS tools (soil conditioning index and soil and water eligibility tools) developed to estimate conservation outcomes. It introduces the new Conservation Management tool that will be used to implement the Conservation Stewardship Program.
Introduction: Goals and Approach of Organic Standards and NRCS Programs
Implementation Varies by State
Organic Concerns About NRCS Enrollment Tools
Tool Performance in the Windsor Organic Research Trial
Additional Resources
References and Citations
The National Organic Program (NOP) and the Natural Resource Conservation Service (NRCS) share the goal of natural resource protection. The NOP defines organic production as “production system that is managed in accordance with the Act and regulations in this part to respond to site-specific conditions by integrating cultural, biological, and mechanical practices that foster cycling of resources, promote ecological balance, and conserve biodiversity.” Promotion of ecological balance and conservation of biodiversity are defining principles of organic agriculture. The NOP requires that organic producers must maintain or improve the natural resources of the operation, including soil and water quality, and minimize soil erosion. Organic growers comply with these requirements by implementing conservation practices, such as crop rotations, cover crops, grass waterways, and contour strips. Many also grow annual and perennial flowering plants (farmscaping) to provide food and habitat for pollinators, natural enemies of insect pests, and other beneficial organisms. Some also erect bird and bat houses to enhance biodiversity and improve pest control.
The NRCS is a Federal agency that pursues natural resource protection goals through the delivery of technical assistance to land owners and attempts to tailor this information to address client needs. The institution administers federal conservation programs that provide cost share and technical assistance for conservation implementation, and, in some cases, financial incentives. Participation in NRCS programs is voluntary and only people managing private lands are eligible. Resource concerns of the agency include: soil quality, water quantity and quality, air quality, production animal and wildlife management, and plant health and suitability. The NRCS maintains the Organic Initiative Practice List and National Organic Program Rules Matrix (PDF; 52KB) which correlates the organic system plan and organic certification requirements with NRCS conservation practices. (Not all practices are appropriate in all areas.) It provides guidance on land management practices as varied as Aquaculture Ponds, Channel Stabilization, Pest Management, Residue and Tillage Management, Conservation Rotation, and Cover Crops for croplands, forests, and pastures. The NRCS standards and practice criteria are fully compatible with organic farming systems and can be readily applied to, and adopted on, organic farms. National practice standards are reviewed and updated every five years (or sooner, if warranted by technology change).
Both the NOP and NRCS approaches appreciate the site-specific nature of farming and conservation concerns. NRCS programs vary across the country because state offices are asked to modify national standards to make them more applicable to their local conditions. States may also propose new standards, introduced as interim standards, that upon review may become national standards after three years. Job Sheets and Technical Notes provide specifics about how to implement practice standards.
USDA provides information about relevant practices and resources specifically for organic systems.
Implementation Varies by State
The NRCS programs and National Organic Program regulations both assess management practices, such as rotation, fertilizer additions, and tillage, as a way to evaluate stewardship. In some cases, NRCS uses producer records of practices to run models or evaluation tools that predict outcomes such as erosion, changes in organic matter, water quality, and biodiversity. Self-reporting of practices, with later field visits for verification, is also used. Organic farmers also depend on self-reporting of practices in the form of a farm management plan, called the Organic System Plan (OSP), that is a required part of their certification document. As a part of this document, organic farmers describe the practices they use to prevent runoff, manage water movement onto their farm, and prevent nutrients from leaving the farm. In addition, organic farmers are required to monitor their practices to demonstrate compliance, and maintain records of all inputs, activities, and transactions; these are examined at least annually during the certification inspection. For more on organic certification documents, see Organic Certification of Vegetable Operations.
The retains and expands several areas of support for organic. This includes support provide through the Environmental Quality Incentives Program (EQIP) in the form of payments and technical assistance for conservation practices, and for transitioning an operation to organic production, and through cost share
Organic Concerns About NRCS Enrollment ToolsThe Conservation Security Program (CSP) was first introduced in the 2002 Farm Bill. Working lands provisions provided an opportunity to reward land managers already implementing conservation practices with "green payments". The initial roll out of the CSP program caused some concern by organic farming groups that felt the program was biased against organic growers because the initial enrollment tool, called the Soil Conditioning Index (SCI), tracked tillage disturbance as a primary factor in reducing soil quality. Many producers who farm organically or live in the warmer climates have had difficulty meeting the minimum criteria for eligibility despite their strong conservation efforts. NRCS has followed up by placing greater emphasis on soil building activities, such a cover crops, mulching, compost additions and by providing expanded training for field agents evaluating practices and giving credit for improving soil biodiversity, which aides nutrient cycling, breaks pest cycles and contributes to increase soil structure, by giving credit for reduced pesticide use and diverse rotations.
Efforts to improve new tools and evaluate performance on organic farms are underway as NRCS works to implement the revised Conservation Stewardship Program contained in the 2008 Farm Bill. The 2008 Farm Bill made many improvements that should be attractive to organic farmers. One key difference is that it seeks to reward producers who have historically used good stewardship practices as well as provide iincentives for those who want to do more. It is no longer watershed based and considers the managment of the farm as a whole, not just individual fields. The new Conservation Stewarship Program will include a crosswalk to help organic and specialty crop producers access the program, as well as outreach and technical assistance to organic and specialty crop producers. It also includes a mandate for NRCS to establish a standard of care for soil, water, and biodiversity that will be predicted based on management practices. NRCS must now establish quality criteria to establish the minimum treatment level necessary to adequately address identified resource concerns for a particular land area. These criteria are described in NRCS’s Field Office Technical Guide. While there are a set of national criteria, each state may add to the criteria to make them more relevant to local conditions.
Tool Performance in the Windsor Organic Research TrialThe NRCS is working to develop and validate tools used to decide which farms—including organic farms—are eligible for program participation. This section reviews some tools that have been used and introduces the new CMT. New tools will build on existing resources including the Soil Conditioning Index (SCI), which evaluates management practices and their influence on soil status. The SCI is currently embedded in RUSLE2 (Revised Universal Soil Loss Equation 2). RUSLE2 a detailed process model that predicts long-term, average-annual erosion by water. The SCI focuses on organic matter and is computed with a weighted function:
SCI = (Organic Matter x 0.4) + (Field Operations x 0.4) + (Erosion x 0.2)
where:
Organic Matter accounts for organic material additions, biomass produced, and decomposition;
Field Operations represents physical disturbance from field operations; and
Erosion is the estimated loss of soil material by sheet, rill, irrigation and/or wind erosion.
When the SCI score is negative, soil organic matter is predicted to be decreasing; for zero or positive SCI scores, soil organic matter is predicted to be stable or increasing. For the history of this tool see Zobeck et al. (2007).
The SCI is estimated for a particular field by running the RUSLE2 model. Users provide information about the soil type and location of the field. Evaluations by NRCS suggest that tool failures often result from problems with model calibration or simple user error. NRCS is working on new expert systems, including the Soil and Water Eligibility Tool (SWET) and products derived from this tool. SWET evaluates management practices based on their contribution to each soil function or water quality concern. The soil properties that SWET scores include organic matter, nutrient cycling, soil habitat, physical stability, and moisture management. The water quality properties SWET scores are sediment, salinity, and surface and groundwater pesticides, nitrogen, and phosphorus. A new conservation compliance tool that is based on SWET is now under development and will be used to determine eligibility for future NRCS programs. Fig. 2 shows the entry screen for this prototype tool. An expert system will be used to determine what practices meet the standards of care established for soil and water resources. Assumptions about practice impacts on soils predict outcomes for soil (organic matter, nutrient cycling, soil physical condition, soil biotic habitat, and soil moisture salinity management) and water quality (leached N, P, and pesticides; sediment loss). Initial validation efforts have compared tool results with measured soil quality data from medium- and long-term research plots (Wienhold et al., 2007; Zobeck et al. 2007).
Figure 2. Screen shot from the SWET tool. The NRCS has already adapted this and incorporated it into a new Conservation Management Tool (CMT).
Table 1 shows the results from an evaluation of NRCS tools (SWET and SCI) and compares them with the soil organic carbon (SOC) concentrations actually measured in the surface soils of replicated research plots in an organic transition experiment in Champaign, IL. Three transitional cropping systems (intensive vegetable, row crop, and perennial pasture or ley), each with three approaches to fertility (crop and cover crop residues only, and residues supplemented with either manure or compost additions), were compared during 2003–2005. For all treatment combinations, the SOC levels increased slightly during the study compared to baseline samples taken at the beginning of transition.
Table 1. Soil quality characterization after three years under different cropping system and soil amendment treatments. SWET2 SCI3 Year 3 SOC Treatment1 Score Eligible? Score Eligible? (%)4 VEG 44 no -0.46 no 2.45 ± 0.72 VEG + manure 52 no 0.86 yes 2.36 ± 0.80 VEG + compost 52 no 2.80 yes 2.39 ± 0.63 ROW 64 yes -0.12 no 2.17 ± 0.41 ROW + manure 72 yes 1.60 yes 2.28 ± 0.58 ROW + compost 72 yes 3.60 yes 2.37 ± 0.40 LEY 101 yes 0.98 yes 2.50 ± 0.31 LEY + manure 109 yes 2.40 yes 2.55 ± 0.38 LEY + compost 109 yes 3.70 yes 2.24 ± 0.63
1 Cropping system and soil amendment treatment. VEG = diversified vegetable rotation; ROW = grain and oilseed rotation; LEY = perennial grass and legume forage.
2 SWET - Soil and Water Eligibility Tool
3 SCI - Soil Condition Index
4 Soil organic carbon, mean ± s.d., n=4
The SWET scores in Table 1 suggest the more-intensively managed vegetable cropping system would degrade soil and water quality, and thus be ineligible for CSP participation. Measurements of SOC suggest this prediction would be false at this site. SWET scores tended to be lower for scenarios without organic matter additions but not enough to change eligibility status based on SWET. Manure and compost addition made a bigger difference in the SCI predictions. Negative SCI scores for the vegetable and row cropped systems without supplemental manure or compost additions predicted a decline in soil organic matter that would have made them CSP ineligible. SOC measured indicate organic matter levels held even where cover crops were the sole source of fertility. In our comparison, both SWET and SCI appeared to overvalue the benefits of supplemental organic matter (particularly compost) additions and the use of perennial pasture and undervalue the benefits of cover crops, particularly in the vegetable cropping system.
Additional Resources- To learn more about EQIP, transitioning to organic agriculture, RC&D's and the additional assistance available from NRCS, contact your local USDA Service Center or visit http://www.nrcs.usda.gov/ (verified 10 March 2010).
- 2008 Farm Bill Side-by-Side [Online]. United States Department of Agriculture—Economic Research Service. Available at http://webarchives.cdlib.org/sw1vh5dg3r/http:/ers.usda.gov/FarmBill/2008/ (verified 10 March 2010).
- Conservation Stewarship Program [Online]. United States Department of Agriculture—Natural Resources Conservation Service. Available at: http://www.nrcs.usda.gov/wps/portal/nrcs/main?ss=16&navid=100120300000000&pnavid=100120000000000&position=SUBNAVIGATION&ttype=main&navtype=SUBNAVIGATION&pname=Conservation%20Stewardship%20Program%20|%20NRCS (verified 10 March 2010).
- For more on the Conservation Benefits of Organic Production go to: http://organicecology.umn.edu/archive/category/education-outreach/page/2/
- For more on Farm Beginnings programs see these websites:
- Minnesota Farm Beginnings, http://www.landstewardshipproject.org/farmbeg.html (verified 10 March 2010)
- Stateline Farm Beginnings, http://www.learngrowconnect.org/what/training/stateline (verified 10 March 2010)
- Central Illinois Farm Beginnings, http://illinoisfarmbeginnings.org/central-illinois-farm-beginnings/ (verified 14 June 2019)
- Dakota Rural Action Farm Beginnings, http://www.dakotarural.org/index.php?option=com_content&view=article&id=... (verified 10 March 2010)
- Lake Superior Farm Beginnings, http://landstewardshipproject.org/morefarmers (verified 10 March 2010)
- Hawthorne Valley Farm Farm Beginnings, http://www.hawthornevalleyfarm.org/education/farmbeginnings.htm (verified 10 March 2010)
- Farm Beginnings Nebraska, http://www.nebsusag.org/farmbeginningsnebraska.htm (verified 10 March 2010)
- Anonymous. 2008. Incentive payments available to new organic farmers. High Plains Journal, Dodge City, KS. 27 Aug. p. 5-B. (Available online at: http://www.hpj.com/archives/2008/sep08/sep1/Incentivepaymentsavailablet.cfm?) (verified 10 March 2010).
- Behar, H. 2008. Organic certification cost share and other 2008 farm bill programs. Organic Broadcaster. Nov/Dec. (Available online at: http://www.mosesorganic.org/attachments/broadcaster/other16.6costshare.html) (verified 10 March 2010).
- Pope, J. 2008. NRCS and RC&Ds help advance organic agriculture across the state. NebNews: the Newsletter of the Nebraska Natural Resources Conservation Service. Summer. p. 3. (Available online at: ftp://ftp-fc.sc.egov.usda.gov/NE/Outgoing/News/nebnews/Summer2008.pdf (verified 10 March 2010).
- Wienhold, B. J., J. L. Pikul, Jr., M. A. Liebig, M. M. Mikha, G. E. Varvel, J. W. Doran, and S. S. Andrews. 2006. Cropping system effects on soil quality in the Great Plains: Synthesis from a regional project. Renewable Agriculture and Food Systems 21: 49–59. (Available online at: http://dx.doi.org/10.1079/RAF2005125) (verified 11 March 2010).
- Zobeck T. M., J. Crownover, M. Dollar, K. F. Bronson, and D. R. Upchurch. 2007. Investigation of Soil Conditioning Index values for southern High Plains agroecosystems. Journal of Soil and Water Conservation 62: 433–442.
This is an eOrganic article and was reviewed for compliance with National Organic Program regulations by members of the eOrganic community. Always check with your organic certification agency before adopting new practices or using new materials. For more information, refer to eOrganic's articles on organic certification.
eOrganic 2842
Managing Manure Fertilizers in Organic Systems
eOrganic author:
Michelle Wander, University of Illinois
This article reviews the basics for manure management in organic systems. Topics covered include National Organic Program regulations, the risk of contaminants in manures, guidelines on how to manage nutrients in manure, and testing manure or compost. Some of the challenges of nutrient supply and test interpretation associated with the repeated use of manures are discussed along with tips and tools you might use to determine manure application rates.
- Introduction, Rules, and Concerns
- Manure Handling: Raw Stacked or Composted
- Managing Nutrients in Manure
- References and Citations
- Additional Resources
Livestock manure is a key fertilizer in organic and sustainable soil management. Manure provides plant nutrients and can be an excellent soil conditioner. Properly managed manure applications recycle nutrients to crops, improve soil quality, and protect water quality. It is most effectively used in combination with crop rotation, cover cropping, green manuring, liming, and the addition of other natural or biologically-friendly fertilizers and amendments.
Use of manure imported from conventional farming operations is allowed by National Organic Program (NOP) standards. There are, however, application restrictions. Manure may only be used in conjunction with other soil-building practices and be stored in a way that prevents contamination of surface or ground water. Many certifiers specify that manure application must not exceed “agronomic application rates”, which means the amount applied must be less than or equal to the requirements of the crop. Manure cannot be applied when the ground is frozen, snow-covered, or saturated.
The NOP regulation (§205.203(c)(1)) specifies that "raw" fresh, aerated, anaerobic, or "sheet composted" manures may only be applied on perennials or crops not for human consumption, or such uncomposted manures must be incorporated at least four months (120 days) before harvest of a crop for human consumption, if the crop contacts the soil or soil particles (especially important for nitrate accumulators, such as spinach). If the crop for human consumption does not contact the soil or soil particles (e.g. sweet corn), raw manure can be incorporated up to 90 days prior to harvest. Biosolids, sewage sludge, and other human wastes are prohibited. Septic wastes are prohibited, as well as anything containing human waste.
Composted plant and animal manures (§205.203(c)(2)) are those that are produced by a process that: (i) established an initial C:N ratio of between 25:1 and 40:1; and (ii) maintained a temperature of 131°F to 170°F for 3 days using an in-vessel or static aerated pile system; or (iii) a temperature of between 131°F and 170°F for 15 days using a windrow composting system, during which period, the materials must be turned a minimum of five times. Alternatively, acceptable composts must meet the November 9, 2006 NOSB Recommendation for Guidance Use of Compost, Vermicompost, Processed Manure and Compost Tea that identifies materials and practices that would be acceptable under 205.203(c)(2). For more information see Making and Using Composts in Organic Systems.
Processed manures are addressed in section §205.203(c)(3). Heat-treated, processed manure may be used as a supplement to a soil-building program, without a specific interval between application and harvest. Producers are expected to comply with all applicable requirements of the NOP regulation with respect to soil quality, including ensuring the soil is enhanced and maintained through proper stewardship.
According to the NOP's July 17, 2007 ruling, “processed manure products must be treated so that all portions of the product, without causing combustion, reach a minimum temperature of either 150°F (66°C) for at least one hour or 165°F (74°C), and are dried to a maximum moisture level of 12%; or an equivalent heating and drying process could be used." To achieve equivalency status, processed manure products can not contain more than 1x10³ (1,000) MPN (Most Probable Number) fecal coliform per gram of processed material sampled and not contain more than 3 MPN Salmonella per 4 gram sample of processed manure.
As always, organic vegetable growers should get label information and check with their certifiers before using purchased compost or processed manure products. See Can I Use This Input On My Organic Farm? for more information.
Some manures are contaminated with hormones, antibiotics, pesticides, disease organisms, heavy metals, and other undesirable substances. Many of the organic compounds, pathogens, protozoa, or viruses can be eliminated through high-temperature aerobic composting. Caution is advised, however, as some disease causing agents, e.g. Salmonella and E. coli bacteria, may survive the composting process. Manure and compost testing is available through commercial labs and is recomended in situations where there is any doubt about the purity of manures. Manure testing is required by the European Union and Canadian standards. The possibility of transmitting human diseases discourages the use of fresh manures and even some composts as pre-plant or sidedress fertilizers on vegetable crops. Apply animal manures at least 90 or 120 days, as applicable, prior to harvest of any crop that could be eaten without cooking.
Best management practices recommended for manure are as follows:
- Avoid manuring after planting a crop to be harvested.
- Incorporation before planting is recommended.
- Do not use dog or cat (fresh or composted) because these species share many parasites with humans.
- Wash all produce from manured fields thoroughly before use.
Cautions or concerns include the following:
- Manures imported from conventional farms can contain residues from hormones or pesticides. (For more information, see Antibiotics and Hormones in Animal Manure Webcast.)
- In rare cases, carryover of persistent herbicides can occur. Most herbicides break down rapidly after application or during normal composting. However, some of those in the pyridine carboxylic acid group such as clopyralid, which is commonly used on grass lawns, break down slowly, even during composting, and are not degraded when ingested by animals because they pass into the urine quickly. Application of manures or composts derived from grass treated with clopyralid is restricted during the “growing season of application” for all farms, not just those that are organic.
- Heavy metals (e.g., As, Cu, and Zn) are fed to livestock and then added to soils in the form of manures. Unlike sludge, metal content does not influence manure application rates to soils but should be considered as metals persist in the soil and will accumulate with repeat application. Concerns over heavy metals, other chemical contaminants, and salinity are most often raised in association with poultry litter. Under federal organic standards, certifiers may require testing of manure or compost if there is reason to suspect high levels of contamination.
- Weed seeds and plant diseases can be effectively controlled by high temperature aerobic composting of manures.
The NOP regulation also requires that manure and other fertility inputs must be managed so that they do not contribute to contamination of crops, soil, or water by excess nutrients, pathogens, heavy metals, or residues of prohibited substances. Whether animals are raised on farm or manures are imported, organic farmers are likely to need to store manure on farm prior to application. Proper manure storage conserves nutrients and protects surface and groundwater. Storing manure can be as elaborate as keeping it under cover in a building, or as simple as covering the manure pile with a tarp. The important point is keeping the pile covered and away from drainage areas and standing water. The storage location should also be convenient to your animals and crop production.
When you are looking for organic forms of nutrients for crop production, manure and manure composts are two of the logical choices. Composting is more than just piling the material and letting it sit. Composting is the active management of manure and bedding to aid the decomposition of organic materials by microorganisms under controlled conditions. Weed and disease problems associated with raw manures can be alleviated with proper composting. Use of composted manures can also reduce P transport to waterbodies (Evanylo et al. 2008).
Organic producers making their own compost must keep records of their composting operation to demonstrate that the compost was produced according to the definition cited above. If the compost is purchased, the grower should ask for documentation from the supplier showing that the compost meets NOP requirements. Keep this documentation, along with purchase receipts, with your other records. If the compost is 100% plant-based, without any animal excrement or by-products, there is no requirement for heating or turning.
Table 1. Comparison of composted and raw manures (from Bary et al., 2000). Compost Manure slow release form of nutrients usually higher nutrient content easier to spread sometimes difficult to spread lower potential to degrade water quality higher potential to degrade water quality less likely to contain weed seeds more likely to contain weed seeds reduced pathogen levels (e.g. salmonella, E. coli) potential for higher pathogen levels higher investment of time or money lower investment of time or money more expensive to purchase less expensive to purchase fewer odors (although poor composting conditions create foul odors) odors sometimes a problem improves soil tilth improves soil tilth Managing Nutrients in Manure
Manure nutrient contents are highly variable and growers must be able to understand and reduce this variability to make the best agronomic and environmental use of these resources. Manure must be carefully managed to prevent over- or under-application and to account for the cumulative environmental effects of application as well as storage. Balancing crop nutritional needs with manures is an ongoing challenge. Finding out about manure composition is critical to its efficient use. Applying too little can lead to inadequate crop growth because of lack of nutrients. Over-application can reduce crop quality and increase the risk of plant diseases. Over-application will also increases the risk of contaminating surface or groundwater.
There are three main sources of variability and uncertainty when using manure:
- Nutrient and moisture content of the manure.
- Material heterogeneity and application variability.
- Availability of nutrients to crops.
Figure 1. Nutrient flow from manure resources to storage facilities and then to field. Nutrients can be lost from all locations but only those arriving on the field have the chance to feed plants. Figure credit: Michelle Wander, University of Illinois.
Manure composition varies with the species of animal, feed, bedding, and manure storage practices. Table 1 shows typical published values for livestock manure. These values may not accurately represent your situation. Nutrient values can vary by a factor of two or more from the values listed in Table 2. This is why it is important to test materials applied instead of guessing.
Table 2. Typical nutrient content of manure (from Koelsch and Shapiro, 2006). Because of variability between farms, individual manure analysis is preferable to the estimates below. % Dry Matter Ammonium–N Organic–N P2O5 K2O Slurry Manure (lb. of nutrient per 1,000 gallons of manure) Dairy 8 12 13 25 40 Beef 29 5 9 9 13 Swine (finisher, wet-dry feeder) 9 42 17 40 24 Swine (slurry storage, dry feeder) 6 28 11 34 24 Swine (flush building) 2 12 5 13 17 Layer 11 37 20 51 33 Dairy (lagoon sludge)* 10 4 17 20 16 Swine (lagoon sludge) 10 6 16 48 7 Solid Manure (lb. of nutrient per ton of manure) Beef (dirt lot) 67 2 22 23 30 Beef (paved lot)* 29 5 9 9 13 Swine (hood barns) 57 4 13 20 Dairy (scraped earthen lots) 46 3 14 11 16 Broiler (litter from house) 70 15 60 27 33 Layer 40 18 19 55 31 Turkey (grower house litter) 70 15 30 Liquid Effluent from lagoon or holding pond (lbs. of nutrient per acre-inch) Beef (runoff holding pond) 0.25 71 8 47 92 Swine (lagoon) 0.40 91 45 104 189 Dairy (lagoon) 2 317 362 674 1082
Value based upon ASAE, 2005, D384.2; Manure Production and Characteristics with exception of those marked with an "*".
Manure Sampling and TestingCommercial laboratories can measure the nutrients in manure and save you from guessing based on table values. Testing laboratories typically charge from $30 to $60. It is important to use a laboratory that routinely tests animal manure, as they will know the correct type of analysis to use. Extension offices can provide you with publications that list manure testing laboratories in most regions; for example, see the Minnesota Department of Agriculture's listing of manure testing laboratories certified for 2009.
A nutrient analysis is only as good as the sample you take. The best time to sample by far is right before you apply the material because N loss in storage is accounted for. Also, if you use manure repeatedly from the same source, you can develop a running average analysis of that manure (over a 3+ year period). A running average is more likely to be accurate than a single sample taken from a storage pile or lagoon. Samples must be fresh and representative of the manure. Follow these steps:
- Ask the laboratory what type of containers they prefer and make sure the laboratory knows when your sample is coming. Laboratories should receive samples within 48 hours of collection. Plan to collect and send your sample early in the week so the sample does not arrive at the lab on a Friday or a weekend.
- If you have a bucket loader and a large amount of manure, use the loader to mix the manure before sampling.
- Take 10–20 small samples from different parts and depths of the manure pile to form a composite sample. The composite sample should be about 5 gallons. The more heterogeneous your pile, the more samples you should take.
- With a shovel or your hands thoroughly mix the composite sample. You may need to use your hands to ensure complete mixing. Wear rubber gloves when mixing manure samples with your hands.
- Collect about one quart of manure from the composite sample and place in an appropriate container.
- Freeze the sample if you are mailing it. Use rapid delivery to ensure that it arrives at the laboratory within 24–48 hours. You can refrigerate the sample if you are delivering it directly to the lab.
Figure 2. Example of a manure analysis report. Note, the report includes "additional information" about the relative value of nutrients which is subject to change. By convention, available nutrient contents are expressed in terms of reference materials used in fertilizer labels.
Laboratories report results on an as-received or a dry weight basis. As-received results usually are reported in units of lb/ton, while dry weight results usually are reported in percent, ppm, or mg/kg. The “as-received” results, as shown above, are easily used to determining application rates. Dry-weight results can be used to compare analyses over time and from different manure sources.
To convert manure analyses reported on a dry-weight basis (in percent) to an as-received basis (in lb/wet ton), multiply by 20 to convert the dry weight percent to lb/ton; then multiply by the decimal equivalent (23%/100) of the solids content.
Example: For beef manure at 23% solids and 2.4% nitrogen (N) on a dry weight basis:
Step 1. 2.4% x 20 = 48 lb N/ton dry weight
Step 2. 48 lb N/ton dry weight x 0.23 = 11 lb N/ton as-is.
Analyses typically include total nitrogen, ammonium nitrogen (NH4+–N), total phosphorus, total potassium, electrical conductivity, and solids (dry matter). If the manure is old or has been composted you may also want to test for nitrate–N. Total carbon (C) and pH are also useful measurements. Total C can be used to determine the C:N ratio and predict whether or not manure addition is likely to cause nitrogen immobilization. Manure with a C:N ratio greater than 25 is likely to 'tie up' or immobilize nitrogen when you apply it to the soil and stimulate a flush of growth by bacteria and fungi. Bedded manures typically have higher C:N ratios.
Manure Application RatesWhen application rates of manure are based on providing adequate nitrogen for crop growth, added phosphorus and potassium levels will often exceed crop need, so manure should not ve the sole N source in an organic system. Excess levels of soil P can increase the amount of P in runoff, increasing the risk of surface water pollution. Many crops can handle high levels of K, but livestock can be harmed by nutrient imbalances if they consume a diet of forages with high K levels. Annual P-based manure or compost application is the most effective method of application when soil P buildup is a concern (Eghball and Power, 1999). Phosphorus-based application rates improve water quality, but reduce the amount of manure applied per area and so increase the land base needed for manure application. Where P buildup is a concern, legumes should be included in the rotation to provide additional nitrogen.
Typically manure is applied before the most N-demanding crop in the rotation and the amount of N likely to be plant available during the year of application is estimated. Nitrogen availability from manure varies greatly, depending on the type of animal, type and amount of bedding, and age and storage of manure (Table 3). Manure contains nitrogen in the organic and ammonium forms. The organic form releases N slowly, while ammonium–N is immediately available for crop growth.
Table 3. Manure N availability in the first year after application (from Bary et al., 2000). Manure type Total N content (%) % available N Broiler litter 4–6 40–70 Laying hen 4–6 40–70 Sheep 2.5–4 25–50 Rabbit 2.5–3.5 20–40 Beef 2–3 20–40 Dry Stack 1.2–2.5 20–40 Separated Solids 1–2 0–20 Horse 0.8–1.6 0–20
Figure 3. This graph can be used to predict N release based on total N content during the first year after manure application. Figure credit: adapted from Bary et al., 2000.
Solid manures contain most of their nitrogen in the organic form, but poultry manure contains substantial ammonium–N and so should not be surface applied to avoid loss of ammonia gas. Poultry and other manures that contain a large proportion of ammonium–N should be tilled into the soil the same day they are spread. Ammonia loss is greater in warm, dry, and breezy conditions where soil pH is high and is reduced in cool, wet weather. The N availability numbers in Table 2 are approximate ranges for each type of manure. Use the lower part of the ranges if ammonia losses are likely, the manure contains large amounts of bedding, or if the measured N content is lower than typical values. Use the upper range if the manure contains little bedding or if the measured N content is high. Expect first year N tie-up from manures containing less than 1% N. Horse manure or other manures with lots of woody bedding may temporarily tie up nitrogen rather than supply nitrogen for crop growth because the wood is still decaying and bacteria that break down the carbon in the wood consume nitrogen. Composting generally reduces the rate of release of manure N by as much as 50% by converting N into more biologically resistant forms.
"Organic Fertilizer Calculators" like the one linked here are extremely useful tools (Gale et al., 2006). Calculators and manure test information are a far better way to calculate application rates than using tables to estimate manure nutrient content and availability. See Additional Resources for more fertilizer calculators.
Monitoring Soil Nutrient LevelsRepeated applications of manure can increase the pool of slow-release nutrients and so the amount of manure needed to meet crop needs will decline over time. Farmers need to reduce the manure application rate for fields that receive repeated manure applications.
Table 4. Effect of 11 years of annual manure additions on the properties of a heavy clay soil planted to continuous corn silage in Vermont (from Magdoff and Van Es, 2009). Original Level Application Rate (tons/acre/year) 0 10 20 30 Soil organic matter (%) 5.2 4.3 4.8 5.2 5.5 CEC (meq/100g) 17.8 15.8 17 17.8 18.9 pH 6.4 6.0 6.2 6.3 6.4 P (ppm) 4 6.0 7.0 14 17 K (ppm) 129 121 159 191 232 Total Pore Space (%) n.d. 44 45 47 50
Table 4 shows trends where repeat application of manure to a clay soil at three rates has influenced soil properties. Organic matter levels were only maintained where rates equaled 20 tons or more. At these rates P and K levels were in excess. To avoid manure-induced imbalances, continually monitor soil fertility, using appropriate soil tests. Use cover crops and lime or other supplementary fertilizers and amendments to ensure soil balance or restrict application levels if needed.
You can use basic soil tests to evaluate the soil for sufficiency or excess of other nutrients. A basic soil test includes P, K, calcium (Ca), magnesium (Mg), boron (B), pH, EC, and a lime recommendation. If you have consistently low levels of P and K and reduced crop growth, you can probably increase your manure application rates. If you have excessive levels of P and K, you should decrease or eliminate manure applications.
Soil tests can be timed to evaluate different aspects of nutrient supply. Testing the year after manures are applied to assess increased P and K supply is recommended (Heming, 2008). Table 5 shows how test levels rise for several years due to the slow release nature of P contained in many manures.
Table 5. The amount of available phosphorus, expressed as fertilizer equivalent P per tonne or m3, made available in years following manure application increases over time and vaires with the type of manure added (from Heming, 2008). Fertilizer equivalent P per tonne Years after application n Median Mean P Cattle FYM 1 39 0.48 0.62 2 55 0.75 1.21 0.006 3 23 1.1 1.82 0.018 4+ 8 2.2 2.61 0.12 Pig manure 1 11 0.44 0.56 2 11 0.93 0.89 0.04 Poultry manure 1 5 1.21 2.44 2, 3 7 5.23 5.89 0.04 Cattle slurry 1 2 -0.37 -0.37 2, 3 5 1.63 2.92 0.05 Pig slurry 1 3 0.82 0.87 2 6 0.97 1.24 0.46 3 3 1.53 1.13 -0.84
P-values are for the mean FEP being the same as at Ct - 1; italics if P < 0.05. Standard errors for each mean are about 0.5 kg/t for FYM and raw cake, 0.2 for pig manure. The local digested cakes contained about 5 kg total P per tonne and raw cake 2.5 kg total P per tonne.
Late season sampling (0–12 inches or more) can be used to determine whether there is surplus nitrate-N remaining in the soil in the fall. If you apply too much manure, unused nitrate N will accumulate. When the fall and winter rains come, the nitrate will leach from the soil and become a potential contaminant in groundwater or surface water. Excess N can also harm some crops, delaying fruiting and increasing the risk of disease damage, freeze damage, and wind damage. Take a "report card" sample as you would any other soil sample, collecting soil cores at multiple spots in the field, and combining the cores together into a composite sample. If late season nitrate–N results are greater than 15–20 mg/kg, you are supplying more N than your crop needs and should reduce or avoid manure application. Report-card nitrate-N levels greater than 30 mg/kg are excessive.
Nutrient requirements for specific crops can be found in Cooperative Extension production guides or from soil test recommendations. Crop performance can be used to help fine tune application rates.
References and Citations- Agricultural Marketing Service—National Organic Program [Online]. United States Department of Agriculture. Available at: http://www.ams.usda.gov/nop/ (verified 10 March 2010).
- Bary, A., C. Cogger, and D. Sullivan. 2000. Fertilizing with manure. PNW0533. Pacific Northwest Extension Publications, Washington State University, Pullman. (Available online at: https://pubs.wsu.edu/ItemDetail.aspx?ProductID=14941&SeriesCode=&Categor...) (verified 10 March 2010).
- Evanylo, G., C. Sherony, J. Spargo, D. Starner, M. Brosius, and K. Haering. 2008. Soil and water environmental effects of fertilizer-, manure-, and compost-based fertility practices in an organic vegetable cropping system. Agriculture, Ecosystems & Environment 127: 50–58. doi:10.1016/j.agee.2008.02.014
- Gale, E. S., D. M. Sullivan, C. G. Cogger, A. I. Bary, D. D. Hemphill, and E. A. Myhre. 2006. Estimating plant-available nitrogen release from manures, composts, and specialty products. Journal of Environmental Quality. 35: 2321–2332.
- Heming, S. D. 2008. The fertilizer equivalent of phosphorus and potassium in organic manures applied to arable soils. Soil Use and Management 24: 318–322. doi:10.2134/jeq2006.0062
- Koelsch, R., and C. Shapiro. 2006. Determining crop available nutrients from manure. NebGuide G1335. University of Nebraska Extension, Lincoln. (Available online at: http://efotg.sc.egov.usda.gov/references/public/NE/G1335_Determining_Cro...) (verified 12 March 2014).
- Kuepper, G., 2003. Manures for organic crop production [Online]. ATTRA Publication #IP127. National Sustainable Agriculture Information Service. Available at: https://attra.ncat.org/product/manures-for-organic-crop-production/ (verified 14 June 2019).
- Magdoff, F., and H. Van Es. 2009. Building soils for better crops. 3rd ed. Sustainable Agriculture Network Handbook Series Book 10. National Agricultural Laboratory, Beltsville, MD. (Available online at: http://www.sare.org/publications/bsbc/bsbc.pdf) (verified 10 March 2010).
- United States Department of Agriculture. 2000. National organic program: Final rule. Codified at 7 C.F.R., part 205. (Available online at: http://www.ecfr.gov/cgi-bin/text-idx?SID=a6a0935ddf00e166695f4c2138bd58d...) (verified 10 March 2010).
- Andrews, N. and J. Foster. 2007. Organic Fertilizer Calculator: A tool for comparing the cost, nutrient value, and nitrogen availability of organic materials. EM 8936-E. Oregon State University Extension Service, Corvallis. (Available online at: http://smallfarms.oregonstate.edu/organic-fertilizer-calculator) (verified 10 March 2010).
- Certified Manure Testing Laboratories [Online]. Minnesota Department of Agriculture. Available at: http://www2.mda.state.mn.us/webapp/lis/manurelabs.jsp (verified 10 March 2010).
- Manure Nutrient Availability Calculator [Online]. Manure Management Planner. Purdue Research Foundation, West Lafayette, IN. Available at: http://www.agry.purdue.edu/mmp/webcalc/nutAvail.asp (verified 10 March 2010).
- Manure Plant Available Nitrogen (PAN) Calculation [Online]. Resources for Nutrient Management Planners. University of Missouri Extension, Columbia. Available at: http://nmplanner.missouri.edu/tools/pan_calculator.asp (verified 10 March 2010).
- Seal of Testing Assurance [Online]. U.S. Composting Council. Available at: http://www.compostingcouncil.org/programs/sta/ (verified 10 March 2010).
- Sullivan, D. M. 2008. Estimating plant-available nitrogen from manure [Online]. EM 8954-E. Oregon State University Extension, Corvallis. Available at: http://ir.library.oregonstate.edu/xmlui/bitstream/handle/1957/20528/em89... (verified 12 March 2014).
This is an eOrganic article and was reviewed for compliance with National Organic Program regulations by members of the eOrganic community. Always check with your organic certification agency before adopting new practices or using new materials. For more information, refer to eOrganic's articles on organic certification.
eOrganic 3132
Organic Farming and Soil Health in the Western U.S. Webinar Series
The Organic Farming Research Foundation and eOrganic presented eight free webinar trainings on organic farming and soil health in the Western U.S. The webinars target agricultural professionals including Extension personnel, other agency personnel, and agricultural consultants in an effort to increase expertise in organic practices that promote soil health. This series is a perfect complement to the farmer guides OFRF has produced on organic soil health practices, available here. The goal of the trainings is to address the need for region-specific resources and knowledgeable Extension services related to organic soil health, biology, nutrient cycling, and more! Recordings, slides and additional presentation notes are available below.
About the PresenterDr. Mark Schonbeck of the Organic Farming Research Foundation will be joined by experts in the Western Region to review the most recent soil health research and practices relevant to the Western Region. The webinars will provide an overview of the topic, best practices for the Western Region, in-depth analysis of the latest research, and an extensive question and answer session after each presentation.
October 24, 2018: Ecological Nutrient Management for Organic Production in the Western RegionThis webinar will explore the role of the soil food web in nutrient cycling and provisioning, and practical strategies for optimizing the availability of limiting nutrients, such as nitrogen, for soil health, organic crop production, and water quality. We will summarize recent research on nitrogen management for organic vegetable and strawberry production in maritime, Mediterranean, and semiarid climates.
Slide handout, Presentation Notes, Recording link: https://www.youtube.com/watch?v=hkXBexLSefk
November 21, 2018: Ecological Weed Management for the Western RegionIn this webinar we will focus on integrated organic weed management strategies that help desired vegetation outcompete weeds, build soil health, and reduce the need for soil disturbance. In addition, we will summarize outcomes of recent research into organic management of field bindweed and other major weeds of Western Region cropland and rangeland.
Slice Handout, Presentation Notes
January 23, 2019: Practical Conservation Tillage for Western Region Organic Cropping SystemsThis webinar will discuss practical approaches to reducing the adverse impacts of tillage and cultivation on soil life and soil health. We will also cover recent research into newer tillage tools and minimum-till strategies for Western Region organic vegetable, fruit, and field crop production.
Slide handout, Presentation Notes, Recording at https://www.youtube.com/watch?v=KFuO_fFo8ls
February 27, 2019: Selecting and Managing Cover Crops for Organic Crop Rotations in the Western RegionCo-presenter: Eric Brennan, USDA ARS, Salinas, CA.
This webinar will discuss best cover crops, mixes, and management methods for optimum soil health and organic cash crop production in the Western Region. We will explore in greater depth the special challenges that farmers face in adding cover crops to dryland cereal grain rotations and other moisture-limited cropping systems.
Eric is a research horticulturist and lead scientist in organic production at the USDA Agricultural Research Service in Salinas, CA where he has worked since 2001. Eric’s research focuses on vegetable and strawberry production with emphasis on cover crops, weed and soil fertility management, and biological control of insect pests. Eric is passionate about long-term research and effective communication of practical research results to farmers and others.
Slide handout, Presentation notes, Recording at https://www.youtube.com/watch?v=wfaBAqUMuo8
March 27, 2019: Breeding New Cultivars for Soil-enhancing Organic Cropping Systems in the Western Regionwith Jared Zystro of the Organic Seed Alliance and Dawn Thilmany of Colorado State University.
This webinar will summarize plant breeding endeavors toward improved vegetable, specialty grain, and other crop cultivars for organic producers in the Western Region, and practical resources to help organic producers obtain the best available seed varieties for their needs. We will also explore emerging opportunities to develop new cultivars for nutrient and moisture use efficiency, competitiveness toward weeds, and enhanced interactions with beneficial soil biota.
Slide handout, Presentation notes, Recording at https://www.youtube.com/watch?v=auTHGHRUbKk
April 17, 2019: Preparing for Drought: The Role of Soil Health in Water Management in Organic ProductionWith climate change exacerbating water scarcity issues throughout the Western U.S., organic producers urgently need practical information on best irrigation and soil moisture management. This webinar will explore the role of best organic soil health management in water conservation and water quality, with emphasis on practical research outcomes for the Western Region.
Slide handout, Presentation notes, Recording at https://www.youtube.com/watch?v=HucXx2E-99Q
May 29, 2019: Meeting Weather Challenges in the Western U.S.: Organic Practices to Mitigate and Prepare for Climate ChangeSlide handout, Presentation notes, Recording at https://www.youtube.com/watch?v=xxXeLJtWFzs
Co-presenter: Maegen Simmonds
This webinar will explore the capacity of sustainable organic systems to sequester soil carbon, minimize agricultural greenhouse gas emissions, and help organic cropping and livestock operations withstand the impacts of climate disruptions already underway. Our presentation will include a summary of recent research findings and practical implications for the Western Region.
Maegen Simmonds is a soil and ecosystem biogeochemist, modeler, and data scientist who works to ensure the long-term sustainability of ecosystems by informing best management practices, decision-making, and policy. Her research focuses on terrestrial ecosystem processes, including land-atmosphere exchange of greenhouse gases (CO2, CH4, N2O). Predicting the interactive effects of climate, wildfire, soil and plant properties, and land management have been central themes in her work.
June 12, 2019: Soil Biology for the Western Region: Organic Practices to Recruit and Nurture Beneficial Biota in the SoilThis webinar will examine the roles of the soil food web and key components thereof in promoting soil health and fertility and sustainable organic crop production. Recent research conducted in organically managed soils in the Western Region will provide the basis for practical guidelines for best soil food web management in organic farming and ranching systems.
Slide handout, Presentation notes.
Funding for this webinar series is being provided by Western SARE.
This is an eOrganic article and was reviewed for compliance with National Organic Program regulations by members of the eOrganic community. Always check with your organic certification agency before adopting new practices or using new materials. For more information, refer to eOrganic's articles on organic certification.
eOrganic 27448
Composting to Reduce Weed Seeds and Plant Pathogens
eOrganic author:
Ed Zaborski, University of Illinois at Urbana-Champaign
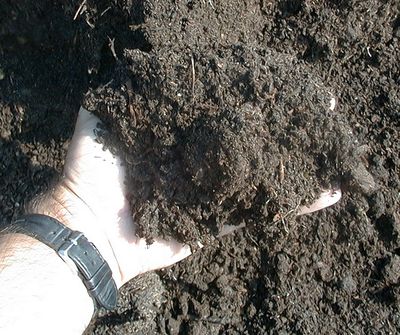
A key feature of organic farming systems is the utilization of organic residues as soil mulches and amendments in an integrated system to maintain and improve soil quality. Organic residues used for these purposes may be produced on the farm, or they may be imported from off-farm sources. Often, fresh organic residues produced in place are used in these practices, such as when cover crops are plowed down as a green manure to build soil organic matter and improve soil fertility, or rolled as a mulch in organic no-till systems to suppress weeds, reduce soil erosion, and conserve soil moisture. Organic residues may also be processed before being used to attain desirable qualities, such as when animal manures are composted to reduce volume and improve stability. Regardless of the circumstances, organic residues that are handled incorrectly can introduce otherwise avoidable problems to the farming system. For example, raw cattle manure may contain viable weed seeds and may spread an otherwise isolated weed infestation more broadly across the farm or, if the manure is imported from outside the farm, introduce a weed problem that previously didn't exist. Similarly, plant residues may be infected with pathogens that can infest subsequent crops. This article provides a brief description of the composting process, discusses the use of composting to reduce weed seeds and plant pathogens, and identifies issues that can lead to the failure of composting to reduce weed seeds and plant pathogens.
What is composting?
For the purposes of organic certification, the National Organic Program rule (United States Department of Agriculture [USDA], 2000) defines compost as:
"The product of a managed process through which microorganisms break down plant and animal materials into more available forms suitable for application to the soil. Compost must be produced through a process that combines plant and animal materials with an initial C:N ratio of between 25:1 and 40:1. Producers using an in-vessel or static aerated pile system must maintain the composting materials at a temperature between 131 °F and 170 °F for 3 days. Producers using a windrow system must maintain the composting materials at a temperature between 131 °F and 170 °F for 15 days, during which time, the materials must be turned a minimum of five times."
–7 C.F.R. § 205.2 (2000)
Composting is the controlled management of the normal biological process of aerobic (in the presence of oxygen) decomposition of organic residues by microorganisms such as bacteria, fungi, and actinomycetes. This process is optimized when the various organic residues are mixed to provide certain conditions:
- a balance of energy (carbon, C) and nutrients (primarily nitrogen, N), with an initial C:N ratio of between 25:1 and 40:1
- sufficient—but not excessive—moisture (typically 40–60% by weight)
- sufficient oxygen to support an aerobic environment (typically 5% or more)
- a pH in the range of 6–8
Under these conditions, populations of microorganisms will thrive and organic residues will be decomposed, consuming oxygen and releasing intermediate breakdown products, carbon dioxide, and heat. As the temperature of the pile rises, the community of microorganisms will go through a succession, culminating in thermophilic (heat-loving) organisms at temperatures above 113 °F (45 °C). If the mass of the compost pile is large enough to be self-insulating, temperatures within the pile during this active phase of composting may reach 131–170 °F (~55–70 °C) within 1–3 days. To maintain biological activity and to bring the active phase to completion, temperatures should be monitored and compost moisture and aeration should be maintained. After the most readily decomposable organic matter in the compost is consumed, biological activity will decrease in intensity, and temperatures and oxygen consumption will decline. The compost then enters the curing phase, during which decomposition proceeds more slowly and organic matter is converted to stable humic substances—the finished or mature compost.
See the related article, Making and Using Compost in Organic Agriculture, for more information about composting.
How does composting reduce weed seeds?Several factors contribute to weed seed mortality during composting. In compost systems assembled and managed in accordance with requirements for organic certification, the most important factors are the interaction between weed species, temperature, time, and moisture (Eggley, 1990; Shiralipour and Mcconnell, 1991; Eghball and Lesoing, 2000; Larney and Blackshaw, 2003; Dahlquist et al., 2007). In general, the higher the temperature to which weed seeds are exposed during the active phase of composting, the higher the weed seed mortality. Similarly, the longer the duration of high-temperature exposure, the higher the weed seed mortality. Thus, Dahlquist et al. (2007) estimated that three of the six weed species they examined under controlled laboratory conditions were unaffected by temperatures of 108 °F, but 90% of the seeds of all six species were killed after less than three hours at 140 °F (Table 1). Furthermore, all six species suffered 100% mortality after less than an hour at 158 °F. Similarly, in Texas, Weise et al. (1998) found that, in composting manure at 35% moisture, barnyardgrass, pigweeds, and kochia seeds were killed after three days at 120 °F; Johnsongrass seed was killed with three or more days of exposure at 160 °F; but field bindweed seeds were killed only after seven days at 180 °F.
Table 1. Estimated number of hours required to kill 90% of seeds (after Dahlquist et al., 2007). Temperature (°F) 140 122 115 108 time required to kill 90% of seeds (hours) Annual sowthistle <1.0 2.1 13.3 46.5 Barnyardgrass <1.0 5.4 12.6 unaffected London rocket <1.0 4.0 21.4 83.1 Common purslane 1.3 18.8 unaffected unaffected Black nightshade 2.9 62.0 196.6 340.6 Tumble pigweed 1.1 107.0 268.5 unaffectedSusceptibility of weed seeds to thermal mortality, however, is influenced by the moisture content of the compost; weed seeds in a dry environment are able to survive higher temperatures for longer times than seeds in a moist environment. Some (Egley, 1990; Thompson et al., 1997) have suggested that thermal mortality may be greatest for fully imbibed seeds—seeds that have absorbed water and split their seed coat in the process of germination. In Nebraska, Eghball and Lesoing (2000) showed that adding water to beef manure compost greatly enhanced weed seed destruction; moist compost was faster and more effective at killing cocklebur, morningglory, pigweed, sunflower, velvetleaf, foxtail, smooth brome, and shattercane than dry compost, in part due to higher compost temperatures.
Other factors are thought to contribute to weed seed mortality during composting. Larney and Blackshaw (2003) observed considerable variability in the relationship between temperature exposure in windrows and seed viability for a number of weeds, and concluded that additional factors, such as germination into lethal conditions or pathogen infestation, were contributing to weed seed mortality. Others have implicated plant-toxic compounds that accumulate to sufficiently high concentrations during composting (phenols, ammonium, and acetic acid, for example) in weed seed mortality and suppression of germination (Eghball and Lesoing, 2000; Shiralipour and Mcconnell, 1991).
How does composting reduce plant pathogens?Several factors are known to contribute to the eradication of plant pathogens and nematodes during composting (Noble and Roberts, 2004):
- heat generated during the active phase of the composting process
- the production of toxic compounds such as organic acids and ammonia
- lytic activity of enzymes produced in the compost
- microbial antagonism, including the production of antibiotics and parasitism
- competition for nutrients
- natural loss of viability of the pathogen with time
- the production of compounds that stimulate the resting stages of pathogens into premature germination
Of all these factors, heat generated during the active phase of the composting process appears to be the most important in pathogen destruction.
Bollen et al. (1998) found that only two of 17 plant pathogens investigated—Olpidium brassicae and one form of Fusarium oxysporum—survived when exposed to small-scale static pile composting of infected plant residues, and then only at greatly reduced levels. Thermal mortality during the active phase of composting was found to be the most important factor affecting pathogen destruction.
In California, Downer et al. (2008) found that unturned piles of fresh and aged green waste (note that these piles would not have satisfied organic certification requirements) did not uniformly expose pathogens to lethal temperatures. They recommended that green waste stockpiles should be turned intermittently to mix pile contents and move propagules to a part of the pile where they would be more likely to be killed by heat, microbial attack, or chemical degradation that occurs during active aerobic composting.
What can go wrong?In general, adherence to a composting process that meets the requirements of organic certification should result in substantial—if not complete—destruction of weed seeds and plant pathogens. Incomplete composting, on the other hand, can result in the survival of weed seeds and/or plant pathogens.
Improperly assembled and maintained piles or windrows may not reach high enough temperatures during the active phase of composting for killing all weed seeds and pathogens. Failure to reach adequate temperatures can have several causes:
- Too high a C:N ratio of initial ingredients, too little water, or too little oxygen can inhibit the rate of decomposition, and thus the production of heat.
- Too much water can starve the pile of oxygen and result in anaerobic decomposition.
- Accumulation of toxic products may inhibit fungal and microbial activity, thus slowing the rate of decomposition.
- Too small a pile or windrow may loose heat too quickly to reach adequate temperatures, whereas too large a pile may have inadequate aeration to support aerobic decomposition.
To avoid these problems, assemble raw materials carefully to achieve the proper starting conditions of C:N, moisture, pile porosity, and size; monitor temperature and moisture conditions; and turn/aerate as needed to maintain a biologically active, aerobic environment. Excellent resources providing detailed instructions and guidelines for composting include The Art and Science of Composting (Cooperband, 2002), Composting on Organic Farms (Baldwin and Greenfield, 2009), and On-Farm Composting Handbook (Rynk, 1992).
Temperatures at the edges and surface of compost piles and windrows may not be sufficient to kill weed seeds and pathogens. This is an especially important risk in static piles that are not turned and mixed during the active phase of decomposition, but rely on forced aeration to maintain an aerobic environment. Thorough mixing or turning during the active phase is essential to ensure that all the material achieves elevated temperatures for a long enough period of time to kill weed seeds and pathogens.
Dry heat is less effective than moist heat at killing weed seeds. Ensure that moisture content of the pile or windrow is maintained at 40–60%.
Contamination with soil or uncomposted residues, especially after the active phase of composting has finished, can lead to the reintroduction of weed seeds or plant pathogens. Avoid adding fresh material after the active phase.
Finished compost can become recontaminated with weed seeds if weeds are allowed to grow and go to seed on or adjacent to the pile or windrow. Similarly, compost can become contaminated with vegetative reproductive structures from some weeds—Canada thistle and rhizomateous grasses, for example—if they are allowed to grow on or adjacent to the pile. Keep vegetation adjacent to stored compost mowed short, and tarp piles or windrows to prevent contamination by wind-blown weed seeds. When moving or spreading finished compost, avoid picking up soil or other contaminants from under or around the pile or windrow.
References Cited- Baldwin, K. R., and J. T. Greenfield. 2009. Composting on organic farms. Organic Production Publication Series, Center for Environmental Farming Systems. North Carolina Cooperative Extension Service, Raleigh. (Available online at: http://www.cefs.ncsu.edu/resources/organicproductionguide/compostingfinaljan2009.pdf) (verified 20 March 2010).
- Bollen, G. J. , D. Volker, and A. P. Wijnen. 1989. Inactivation of soil-borne plant pathogens during small-scale composting of crop residues. Netherlands Journal of Plant Pathology 95 (Supp 1): 19–30.
- Cooperband, L. 2002. The art and science of composting. Center for Integrated Agricultural Systems, University of Wisconsin, Madison. (Available online at: http://www.cias.wisc.edu/wp-content/uploads/2008/07/artofcompost.pdf) (verified 20 March 2010).
- Dahlquist, R. M., T. S. Prather, and J. J. Stapleton. 2007. Time and temperature requirements for weed seed thermal death. Weed Science 55:619–625. (Available online at: http://dx.doi.org/10.1614/WS-04-178.1) (verified 17 Nov 2009).
- Downer, A. J., Crohn, D., Faber, B., Daugovish, O., Becker, J. O., Menge, J. A., and Mochizuki, M. J. 2008. Survival of plant pathogens in static piles of ground green waste. Phytopathology 98:547–554. (Available online at: http://dx.doi.org/10.1094/PHYTO-98-5-0547) (verified 29 June 2010).
- Eghball, B, and G. W. Lesoing. 2000. Viability of weed seeds following manure windrow composting. Compost Science & Utilization 8:46–53.
- Egley, G. H. 1990. High-temperature effects on germination and survival of weed seeds in soil. Weed Science 38:429–435.
- Larney, F. J., and R. E. Blackshaw. 2003. Weed seed viability in composted beef cattle feedlot manure. Journal of Environmental Quality 32:1105–1113. (Available online at: http://dx.doi.org/10.2134/jeq2003.1105) (verified 17 Nov 2009).
- Noble, R., and S. J. Roberts 2004. Eradication of plant pathogens and nematodes during composting: A review. Plant Pathology 53:548–568. (Available online at: http://dx.doi.org/10.1111/j.0032-0862.2004.01059.x) (verified 29 June 2010).
- Rynk, R. 1992. On-farm composting handbook. Northeast Regional Agricultural Engineering Service, Ithaca, NY.
- Shiralipour, A., and D. B. Mcconnell. 1991. Effects of compost heat and phytotoxins on germination of certain Florida weed seeds. Soil and Crop Science Society of Florida Proceedings 50:154–157.
- Thompson, A. J., N. E. Jones, and A. M. Blair. 1997. The effect of temperature on viability of imbibed weed seeds. Annals of Applied Biology 130:123–134. (Available online at: http://dx.doi.org/10.1111/j.1744-7348.1997.tb05788.x) (verified 17 Nov 2009).
- United States Department of Agriculture. 2000. National organic program: Final rule. Codified at 7 C.F.R., part 205. (Available online at: http://www.ecfr.gov/cgi-bin/text-idx?c=ecfr&sid=3f34f4c22f9aa8e6d9864cc2683cea02&tpl=/ecfrbrowse/Title07/7cfr205_main_02.tpl) (verified 14 June 2010).
- Wiese, A. F., J. M. Sweeten, B. W. Bean, C. D. Salisbury, and E. W. Chenault. 1998. High temperature composting of cattle feedlot manure kills weed seed. Applied Engineering in Agriculture. 14:377–380. (Available online at: http://amarillo.tamu.edu/files/2010/11/bean6_High-Temperature-Composting.pdf) (verified 29 June 2010).
- Composting | Reduce, Reuse, Recycle | US EPA [Online]. U.S. Environmental Protection Agency. Available at: http://www2.epa.gov/recycle/composting-home (verified 29 Jan 2010).
- Composting. WSU - Center for Sustaining Agriculture and Natural Resources [Online]. Board of Regents, Washington State University. Available at: http://csanr.wsu.edu/pages/BIOAg_Compost (verified 29 August 2011).
- Cornell Composting. Cornell Waste Management Institute [Online]. Cornell University. Available at: http://compost.css.cornell.edu/ (verified 16 Dec 2009).
- LeaMaster, B., J. R. Hollyer, and J. L. Sullivan. 1998. Composting animal manures: Precautions and processing. University of Hawaii, College of Tropical Agriculture and Human Resources. Available online at: http://www.ctahr.hawaii.edu/oc/freepubs/pdf/AWM-1.pdf (verified 24 Nov 2009).
- US Composting Council [Online]. US Composting Council. Available at: http://www.compostingcouncil.org/ (verified 16 Dec 2009).
This is an eOrganic article and was reviewed for compliance with National Organic Program regulations by members of the eOrganic community. Always check with your organic certification agency before adopting new practices or using new materials. For more information, refer to eOrganic's articles on organic certification.
eOrganic 4751
Organic Potting Mix Basics
eOrganic author:
Michelle Wander, University of Illinois
This article covers basic information about organic potting mixes for organic farming systems. It includes an overview covering the kinds of media (compost, peat, and manure-based materials) commonly used, potting mix test information, and information on how to make and use organic potting mixes in your organic greenhouse operation. It also summarizes basic organic potting mix recipes and provides tips on how to handle materials.
OverviewSoil or potting mixes used to start seed must be very light, have high water- and air-holding capacity and be free of disease and weed seeds. Planting mixes containing soil can be blended with peat, humus, compost, and/or manure with approved additives intended as soil conditioners and/or organic fertilizers. The need for disease- and weed-free media favors the use of soilless media, or potting mixes, that are made primarily from natural materials, such as peat, compost, and/or manures augmented with perlite, vermiculite, peat moss, and organic fertilizers. Soil-based mixes provide adequate water holding and supply some nutrients. Loamy soils are usually preferred for soil based media. A mix made with high quality compost will generally provide adequate amounts of phosphate, potash, and trace elements, but in some situations a mix will be need to be supplemented by adding bone meal, rock phosphate, sul-po-mag, greensand, kelp meal, and/or other approved organic fertilizers.
Figure 1. A commercially supplied soilless germination mix containing peat, compost, and perlite. Photo credit: Michelle Wander.
Growers can
- purchase premade mixes,
- arrange for a custom blend made by a media supplier, or
- mix their own media.
Consistency and time savings associated with the purchase of premade mixes as well as their comparative ease of handling, make them an attractive option for many. Freight costs might be a consideration, especially where growers can produce their own high quality compost. Purchased and homemade mixes can vary notably. Typically, media pH ranges from 4.2 to 7.5, with soluble salts from 20 to 840 dS and nitrate from trace to over 800 ppm.
The most important first step for growers is to make sure ingredients are approved for organic production. This means they are approved for organic use, and include “OMRI Listed” or "WSDA Approved" on their label. If you have questions, confirm with your certification agent that any potting mix you are about to use, or any ingredient you intend to use, is allowed. Growing media and commercial composts can include fertilizers, wetting agents, and other inorganic materials not approved, so exert caution. Products like peat moss or limestone are sometimes treated with prohibited materials including wetting or anticaking agents. See Can I Use This Input on My Organic Farm? for more information.
Test Your Mix Before You Use itIf you have time and are unfamiliar with a mix it is a good idea to get a greenhouse soil test (Grubinger, 2007). These typically assess pH, soluble salts (electrical conductivity), and nitrogen in the nitrate and ammonium forms. For the saturated media extract (SME) method, the sample is mixed with distilled water at a standard dilution and then analyzed. When evaluating a mix consider the following (Leonard and Rangarajan, 2007):
Physical properties- Particle size is appropriate to container
- Density, will it hold up plants?
- Good aeration and water-holding capacity
- 10 to 20% air space and 40 to 60% water when moist
- Nutrients levels: low to moderate
- Salts and EC: 4 to 8 dS/m (saturated paste extraction)
- pH near 7
- No plant pathogens
- Decomposition rate should be low
- Organic sources are often inconsistent
- Compost based media: select a well-cured compost
- Some soluble fertilizer sources, such as fish meals, are available
- Some will result in ‘composting’
- Others increase salts
Premium potting mixes will have a pH between 5.5 and 7, soluble salts between 1 and 5 mmhos, nitrate N between 10 and 200 ppm, P2O5 greater than 3 ppm, K2O greater than 25 ppm, Ca and Mg present in amounts greater than 30 and 10, respectively with sodium and chloride contents falling below 130 and 200 ppm. Water-holding capacity should be 65%. Consider the results based on the crop you are growing and how long you want to hold it before planting. Compare results from batches of potting mix that performed well with those that underperformed.
Bioassays, actually growing plants in the mix, are another way to evaluate your potting mix. Cress, oats, beans, and lettuce are fast-growing crops that can be seeded in the mix before a major planting is initiated. Sensitive crops, like onions, which seem to require a very high quality mix for good germination and growth, are also good to grow. It also makes sense to evaluate crops that you grow as part of your bioassay. Note, seeding depth and adequate water and temperature conditions as well as seed quality can confound results.
Making and Using MixesFactors influencing choice of mix and handling procedures include:
- The availability and price of high quality inputs. Availability is a concern as growers need reliable and consistent media. The main ingredients of soilless mixes are bulky and lightweight and so transportation costs are an important consideration. It is important for organic growers that inputs be from renewable sources.
- Grower desire and ability to make their own consistent compost and mix. This requires access to bulky C-rich materials to make appropriate compost and the time and skill required to do this reliably.
- Storage and handling facilities. Purchased mixes can be delivered in bagged or bulk form as desired by the grower. Bulk materials should be stored to preserve or improve quality and this will vary with the age and stage of decay. Bagged materials are easier to handle but more costly.
- The number and types of seed starts or transplants to be produced. Germination mixes will have lower nutrient content than media intended to produce larger plants. Small seeds placed at the surface require small particle size and excellent seed-media contact.
- Green house space available to grow starts and target size or age of ‘finished’ plant. Cell sizes and cell shape interact with potting mix particle size to determine the water-holding and nutrient supply capacity. Larger cells can support plants longer but require more space.
- Watering regime. The containers and mix used must work well with the watering technique and quality of water available to the grower. Consider whether water has a high pH, and/or whether foliar feeding should be considered in management.
- Nutritional needs. Nitrogen (N) availability is usually the biggest challenge to organic potting mix formulation because sources are quite variable. Nitrogen is often insufficient when compost is the sole nutrient source. This is why organic potting mixes are often supplemented with alfalfa meal, blood meal, crab meal, etc. when seedlings are to be grown for several weeks. Phosphorus limitations typically show up in early spring, especially in summer crops like tomatoes, when start house temperatures are too low and limit mineralization. The smaller the cell size the more important that the mix contains adequate nutrients. Liquid feeding with fish emulsion or other soluble organic fertilizers can supplement the plants' nutrient needs, when mixes are exhausted of N. Some growers repot plants in fresh mix, often in larger containers, as a way to keep them growing well.
A variety of organic and inorganic ingredients are commonly used in preparing potting mixes, including:
- Material and Description Compost is derived from the biological decomposition of organic matter accomplished by mixing and piling to promote aerobic and/or anaerobic decay. Composting minimizes pathogens, viable weed seeds, and odors. Composts hold water and provide aeration while providing needed nutrients. The lack of availability of consistent, high-quality compost prevents its widespread use for organic media.
- Characteristics Compost used for potting mixes should have a pH of 6.5–8, less than 0.05 ppm ammonia, 0.2–3 ppm ammonium, less than 1 ppm nitrite-N, less than 300 ppm nitrate-N, and more than 25% organic matter. Finished compost should have soluble salts less than 3 mmohs and moisture content 30–35%. Composts made from manures typically have higher nutrient contents (C:N less than 20) than those made from plant materials where the C:N is higher (20–30).
- Common Usage Compost can be used in place of peats or added as a source of fertility. It commonly makes up 20–70% of media, depending on quality and purpose of use, and often displaces peat in mixes. Typically 75% of mix is made of compost and/or peat with 25% perlite or vermiculite. Quality and proportion varies depending on materials (feedstocks) used to make compost. The proportion of peat to compost should be varied with the qualities of compost. Low C:N materials should be added in smaller proportions.
- Material and Description Dried, pulverized, shredded, composted, or otherwise processed, manipulated, or treated animal manures are the excreta of animals mixed with whatever organic bedding or other materials are needed to follow good dairy barn, feedlot, poultry house, or other livestock practice in order to maintain proper sanitary conditions; to conserve plant food elements in the excreta; and to absorb the liquid portion without the addition of other material.
- Characteristics Ideally, manure should be composted before it is added to mixes. Manures vary greatly in quality; some are very good fertilizers but analysis must be done before you can be sure of this.
- Common Usage Manure may be added in small amounts as a source of fertility. Variability in quality makes its optimal use difficult unless very recent soil tests have been made. In most cases manure will be nutritionally imbalanced and require that additional sources of N be added. If the harvested part of the crop has the potential to come in contact with the manure, soil, or soil particles (for example, by soil splash), manure may not be used less that 120 days prior to harvest for human consumption.
Sphagnum peat moss
- Material and Description Peat is a common ingredient in soilless mixes because it is widely available, relatively inexpensive, and has desirable physical characteristics. It holds a lot of water and air and decomposes very slowly. Most peat used in the U.S. comes from Canada and is harvested at rates that are renewable. Barks—even aged barks—should be avoided as seedling mix components because they immobilize nitrogen.
- Characteristics Peat is quite acidic (pH of 3.5–4.0); limestone is usually added to the mix to balance the pH. Add about 5–15 lb lime per cubic yard for mixes containing 70% peat. Peat is not a source of plant nutrients. Color varies with extent of decay; lighter-colored peats (grower or professional grade) provide more aeration. Finer, darker peats (retail grade) are used as a soil amendment.
- Common Usage Often makes up 30–80% of the mixes. Blending equal parts peat and compost results in desirable pH range.
Perlite
- Material and Description Is a silica-based volcanic rock that has been heated using natural gas, causing it to expand and become less dense. It improves aeration and water holding capacity of mixes.
- Characteristics Perlite is sterile and has a neutral pH.
- Common Usage Typically makes up 30–50% of mixes when combined with peat or high C:N compost.
Vermiculite
- Material and Description Is a micaceous mineral expanded in a furnace using natural gas.
- Characteristics Handled roughly, it can lose its air holding capacity. Vermiculite can supply some K, Mg, and other trace minerals.
- Common Usage Typically makes up 30–50% of mixes when combined with peat or high C:N compost.
Coconut coir
- Material and Description Is a by-product of coconut fiber industry that is renewable but requires transportation from long distances. Coir lasts longer than peat but usually is more expensive due to transportation costs.
- Characteristics Coir has higher pH (5.5&endash;6.8), electrical conductivity and thus, soluble salts than peat. It is easier to wet than peat. Depending on fertilization practices, coir can become acidic.
- Common Usage Can be blended to high proportion of mixes (up to 80% reported in the literature with success). Coir is commonly blended with perlite and compost.
Worm castings
- Material and Description Produced by red worms as they breakdown organic wastes. Inconsistent quality makes custom mixes necessary.
- Characteristics Castings are nutrient rich and claimed to have growth promoting attributes. The amount of available P and N varies with bedding materials.
- Common Usage Often makes up 10–40% of mixes; the proportion varies with composition in the same way as other composts or manures.
Kenaf
- Material and Description This is the waste product of a fibrous plant grown in the southern U.S. Waste of core fibers of kenaf is suitable for use as potting media.
- Characteristics Ideally is composted before use so it does not immobilize nitrogen.
- Common Usage Blended at up to 50% of mixes, it is advocated as a possible peat replacement where available.
Recipes range from simple to complex. Most growers begin with a recipe and quickly make it their own. A challenge associated with many of these recipes is the reporting of components as percents or proportions where it is not clear whether this is by volume or mass. In many cases the recipes include a mixture of volumes and masses to be combined in some proportion that is difficult to determine exactly. Volume can be converted to cubic feet or yards. Some common conversion factors are:
- 27 cubic feet in a cubic yard (3’ x 3 ’x 3’)
- Loose bags come in 1 to 4 cubic feet
- Compressed bales contain 3.8 cubic feet
- Four 2 gallon buckets equal a cubic foot
Figure 2. Soil block mix. Photo credit: Chris Jagger and Melanie Kuegler, Blue Fox farm.
For a potting mix example from an experienced farmer, watch this video of Steve Pincus of Tipi Produce.
For another potting mix example, see gardener Bob Strawn's Potting Mix article describing the mixes he uses in his container gardening.
The following recipes are cited in Kuepper (2004) unless otherwise specified.
Seedling mixes for starting transplantsSeed mix (Biernbaum, 2001)
- 2 parts screened compost
- 4 parts sphagnum peat
- 1 part perlite
- 1 part vermiculite
- Lime as needed to adjust pH to 6
Seed mix - standard soilless (Biernbaum, 2001)
- 50–75% sphagnum peat
- 25–50% vermiculite
- 5 lbs of ground or superfine dolomitic lime per cubic yard of mix
- Blood meal, rock phosphate, and greensand at 5 to 10 lbs per cubic yard
Organic seedling mix (Biernbaum, 2001)
- 10 gallon of 2 year old leaf mold, sifted
- 10 gallons of sifted compost
- 5–10 gallons of sphagnum peat
- 5 gallons of perlite
- 5 gallons of coarse river sand
- 3 cups blood meal
- 6 cups bone meal
Soilless potting mix (used by Windsor Organic Research on Transition project, E. Zaborski)
- 1 part compost
- 1 part vermiculite
- 1 part peat moss
Screened with ¼ inch screen to mix together. Per 1 gallon mix add:
- 0.6 oz blood meal (17.01 grams)
- 0.4 oz clay phosphate (11.34 grams)
- 0.4 oz greensand (11.34 grams)
Soil-based seedling mix (Hamilton, 1993)
- 2 parts loam (stacked turf to kill any weed seed and disease)
- 2 parts sphagnum peat
- 2 parts coarse grit (sand)
- 30 g or 1 oz lime for each 2 gallon bucket (9 liters)
- 60 g or 1 oz blood meal for each 2 gallon bucket (9 liters)
Organic potting mix (credited to Eliot Coleman in Kuepper, 2004).
- 1 part sphagnum peat
- 1 part peat humus (short fiber)
- 1 part compost
- 1 part sharp sand (builder's)
to every 80 quarts of this add:
- 1 cup greensand
- 1 cup colloidal phosphate
- 1½–2 cups crabmeal or blood meal
- ½ cup lime
Soil block mix (Kuepper, 2004; adapted from Coleman, 1995)
- 3 buckets (standard 10-qt. bucket) brown peat
- ½ cup lime (mix well)
- 2 buckets coarse sand or perlite
- 3 cups base fertilizer (blood meal, colloidal phosphate, and greensand mixed together in equal parts)
- 1 bucket soil
- 2 buckets compost
Seedling mix for soil blocks or seedling flats (from John Greenier of Stoughton, WI in Kuepper, 2004)
- 2 3-gal. buckets Sphagnum peat moss
- ¼ cup lime
- 1½ cups fertility mix (below)
- 1½ buckets vermiculite
- 1½ buckets compost
Fertility mix:
- 2 cups colloidal (rock) phosphate
- 2 cups greensand
- 2 cups blood meal
- ½ cup bone meal
- ¼ cup kelp meal
Directions for mixing:
- Add peat to cement mixer or mixing barrel.
- Spread the lime and fertility mix over the peat.
- Mix these ingredients thoroughly.
- Add the compost and vermiculite and mix well again.
- When done, examine the distribution of vermiculite to ensure that it has been mixed in evenly.
Note that all bulk ingredients should be screened through 1/4 inch hardware cloth. Well matured, manure-based compost should be used (avoid poultry manure and wood-chip bedding).
Mixes for larger plants or containersThese mixes require the addition of mined nutrients from natural sources.
Cornell Organic Substitute for Classic Mix (as modified by Biernbaum, 2001)
- ½ cu yd. sphagnum peat
- ½ cu yd vermiculite
- 5 lbs ground limestone
- 2–4 lbs bone meal
- 5 lbs blood meal
Figure 3. Flats can be reused but must be cleaned between uses with approved materials. Photo credit: Michelle Wander, University of Illinois.
- Growers are encouraged to experiment with materials not familiar to them before committing a large number of resources and time to an unknown. The risk of losing an early planning are too great for you to just risk it on an unknown media. Producers growing variety of crops need to consider space and the timing of transplants needed. Crops with similar morphology, germination times, nutrient needs and water requirements should be seeded together.
- If you make your mix, you might let it rest for a period of time before using if you have added ingredients like blood meal or poultry manure that can release ammonia that might damage plants. Many experienced growers advocate that mixes be blended and aged or pre-wetted and left for a week before seeding.
- Containers range in size and form: soil blocks, trays and flats allow producers to grow and handle separate plants that maintain intact root balls for planting; pressed peat pots and soil blocks allow producers to grow plants without using plastics; transplants produced with larger cell size (48 to 72 cells per tray) are easier to grow and maintain.
- Plants in peat or soil blocks can be trasplanted without removal from pots. This reduces damage caused by extraction and separation. Roots ‘air prune’ when they reach the edge of the block. Soil blockers are available for small and large scale growers.
- Seed separation to facilitate precision seeding and reduce thinning can be done with a variety of technologies. Commercial and hand build vacuum seeders or needle seeders are commonly used. Commercial seeders and hand drilled seed plates can be expensive (hundreds of dollars) but, if you plant a lot of flats they can be worth it.
- Before filling containers media should be properly screened and moisture should be adjusted. Store this in air tight containers in the shade. Once peat and other highly organic materials dry out they can be difficult to rewet.
- Flats or trays should be cleaned if reused. Surface disinfestants, such as sodium hypochlorite solutions (household bleach) should be used to clean work surfaces, propagation tools, gloves, boots, and equipment to eliminate pathogens. Keep in mind, however, that most detergents are not allowed in certified-organic vegetable production because they include a synthetic surfactant. Soaps generally are permitted. Prior to using any cleaning products, check with your certifier to make sure they meet NOP standards. For a brand name list of approved products, visit the Organic Materials Review Instistute.
- Screening of materials prior to mixing and before filling cells is important. Common methods include ¼ to ½ inch screen or hardware cloth. Screens are often built for placement on wheel barrows for ease of processing. Larger organic materials removed can be returned to compost or used for larger pot mixes.
- Poor seedling performance may or may not be caused by the media. Potting mix characteristics that may cause poor seedling performance include low levels of available nutrients, high levels of soluble salts and excess nutrients, excessive density of the mix, and/or the presence of compounds like volatile organic acids that can inhibit plant growth. Cultural practices and conditions can influence plant performance too. Over watering, and/or slow growth caused by low temperatures or limited light can weaken plants and make them susceptible to damping off.
- Biernbaum, J. 2001. Organic transplant production for the advanced market gardener. Course materials. Organic University, La Crosse, Wisconsin. 15 March 2001. Midwest Organic and Sustainable Education Service (MOSES), Spring Valley, WI.
- Coleman, E. 1995. The new organic grower: A master's manual of tools and techniques for the home and market gardener. Chelsea Green Publishing Company, White River Junction, VT.
- Grubinger, V. 2007. Potting mixes for organic growers [Online]. University of Vermont Extension, Brattleboro. Available at: http://www.uvm.edu/vtvegandberry/factsheets/OrganicPottingMixes.pdf (verified 4 February 2012).
- Hamilton, G. 1993. The organic gardening book. Dorling Kindersley, New York.
- Kuepper, G. 2004. Potting mixes for certified organic production [Online]. ATTRA Publication #IP112. National Sustainable Agriculture Information Service. Available at: https://attra.ncat.org/product/potting-mixes-for-certified-organic-produ... (verified 12 Jun 2019).
- Leonard, B., and A. Rangarajan. 2007. Organic transplant media and tomato performance. Deptartment of Horticulture, Cornell University, Ithaca, NY. Available online at: http://cwmi.css.cornell.edu/organictransplant.pdf (verified 2 June 2015).
- Jagger, C., and M. Kuegler. 2008. Making soil blocks. Wanna Farm: A Farming Resource Blog. No longer available online.
- Jagger, C. and M. Kuegler.Soilblock recipe. Wanna Farm: A Farming Resource Blog. No longer available online.
- Marlin, J. 2008. Tipi Produce's organic potting soil recipe [Online video]. Agroecology and Sustainable Agriculture Program, University of Illinois, Urbana. Available at: http://www.vimeo.com/2316005 (verified 10 March 2010).
- OMRI - Organic Materials Review Institute [Online]. Available at: http://www.omri.org/ (verified 10 March 2010).
This is an eOrganic article and was reviewed for compliance with National Organic Program regulations by members of the eOrganic community. Always check with your organic certification agency before adopting new practices or using new materials. For more information, refer to eOrganic's articles on organic certification.
eOrganic 3442
Biology and Management of Pickleworm and Melonworm in Organic Curcurbit Production Systems
eOrganic author:
Dr. Geoff Zehnder, Professor, Department of Entomology and Coordinator, IPM & Sustainable Agriculture Programs, Clemson University
Scientific Names: Pickleworm (Diaphania nitidalis); Melonworm (Diaphania hyalinata); Crambidae (formerly Pyralidae); LEPIDOPTERA
This article presents an overview of the biology, behavior and damage associated with pickleworm and melonworm, two closely related insect pests of cucurbit crops. Discussed are management approaches that are appropriate for certified organic farming systems. Although the two species are similar in their biology and distribution, they differ in the damage that they cause to cucurbit crops, with pickleworm generally considered to be the more economically important pest.
Biology, Appearance, and DistributionPickleworm and melonworm are tropical insects, and cannot tolerate cold temperatures. In the U.S., they are known to overwinter only in south/central Florida and southern Texas. The adult moths disperse northward each spring. During the summer, the pickleworm moth migrates into the Carolinas and can move into more northern states and even westward to Oklahoma and Nebraska. The melonworm, however, is rarely found north of the Gulf states.
Figure 1. Adult pickleworm moth. Photo credit: Natasha Wright, Florida Department of Agriculture and Consumer Services, Bugwood.org.
The pickleworm adult is a flashy moth with wide triangular wings and a wingspan of about one inch (Fig. 1). The wings are mostly iridescent brown, with a central band of semi-transparant yellow. The melonworm moth is slightly smaller with white, slightly iridescent wings centrally, and edged with a broad band of dark brown (Fig. 2). Both moths display brush-like appendages at the tip of the abdomen when at rest.
Pickleworm and melonworm moths are generally inactive during the day, but melonworm will fly short distances when disturbed (Smith, 1911). Peak flight activity of pickleworm moths occurs 3–5 hours after sundown (Capinera, 2005).
Moths lay tiny eggs in on growing areas of the plant, such as new leaf buds, flowers and shoots, and eggs generally hatch in 3–4 days. Pickleworm and melonworm can produce as many as four generations per season.
Figure 2. Adult melonworm moth. Photo credit: Alton N. Sparks, Jr., University of Georgia, Bugwood.org.
The younger larvae of pickleworms are thin white caterpillars with numerous small black spots but as they mature they become plump and darker in color, and lose their spots. Larval color in the last instar stage is variable depending on food source; off-white, green, yellow and orange colored larvae can be found. Pickleworm larvae prefer to feed on blossoms initially, and blossom damage can severaly reduce fruit set. When about half-grown, pickleworm larvae often bore into fruit and continue to feed there causing internal damage and producing soft excrement (Fig. 3). Both young and old fruit are attacked, but they prefer young fruit before the rind has hardened. Pickleworm usually pupates within a leaf fold, and dead, dry leaf material is often used.
Figure 3. Pickleworm larvae and excrement inside fruit. Photo credit: Clemson University-USDA Cooperative Extension Slide Series, Bugwood.org.
Newly hatched melonworm larvae lack color, but are pale green in the second instar stage and mature melonworm larvae are dark green with two lateral white stripes (Fig. 4). Melonworm larvae construct a silken structure on the undersides of leaves and continue to develop and feed on foliage. Pupation occurs inside a silken cocoon on the plant, often within a folded section of green leaf material.
Figure 4. Melonworm larvae. Photo credit: Alton N. Sparks, Jr., University of Georgia, Bugwood.org.
These two caterpillars infest only cucurbits; both wild and cultivated species may serve as hosts. Please refer to the Management section below for a listing of resistant varieties.
Though the pickleworm prefers squash (summer squash is highly preferred), it may also cause damage to cucumber and cantaloupe. Generally speaking, varieties of Cucurbita maxima and C. moshata are more resistant than those in the C. pepo species. Therefore, muskmelon, winter squash, and gourd are rarely damaged by pickleworm, and watermelon is also not a preferred host. Pumpkin is a variable host, probably because pumpkins have been bred from several Cucurbita species.
Melonworm commonly infests summer and winter squash. The Cucumis species—cucumber, gerkin, and cantaloupe—are attacked but not preferred, and watermelon is a rare host. Pumpkin is a variable host.
DamageIn their overwintering areas of southern Florida and Texas, crop damage can occur early in the growing season. Both species disperse northward as the season progresses and it regularly takes one or two months for the dispersing moths to move into the Carolinas. Therefore, in the southeastern states north of their overwintering habitats, infestation of cucurbits by pickleworm and melonworm is usually not observed until late June or early July. Early season crops usually escape injury. In some years they may travel further north where infestation of crops may not begin until August or September.
Melonworm damage is mainly on foliage, especially if foliage of a favored host plant is available (Fig. 5). Usually the leaf veins are left intact, resulting in lace-like plant remains. However, if the available foliage is exhausted, or the plant is a less preferred species, then the larva may feed on the surface of the fruit, or even burrow into the fruit (Fig. 6). Thus, growers may refer to these melonworm larvae as rindworms because they cause scars on the surface of melons.
Figure 5. Melonworm damage to foliage. Photo credit: Alton N. Sparks, Jr., University of Georgia, Bugwood.org.
Figure 6. Left: Illustration showing pickleworm life stages and damage. Credit: Art Cushman, USDA; Property of the Smithsonian Institution, Department of Entomology, Bugwood.org.
Right: Pickleworm entrance hole on squash. Photo credit: Alton N. Sparks, Jr., University of Georgia, Bugwood.org.
Unlike the melonworm, the primary concern with pickleworm is damage to the fruit. Young pickleworms usually feed for a time among small leaves at the growing tips of vines or within blossoms. Growing vines sometimes become riddled with holes and cease to grow. Larvae are often found in the squash flowers where they hide under the ring of stamens at the base of flowers. When about half grown, pickleworms bore into the fruit and continue to feed there causing internal damage. Entry holes are usually marked with a pile of white excrement or frass. Both young and old fruits are attacked, but they prefer young fruit before the rind has hardened. After the fruit rind has been punctured the fruit soon rots.
SamplingIt is difficult to detect the presence of pickleworm and melonworm before damage occurs because of their small egg size, and nocturnal flight behavior of the adult moths. Although sex pheromones of both species have been discovered and synthesized, at present pheromone trapping systems are not commercially available. Checking plants for early stages of leaf damage and the presence of larvae are the most effective ways to monitor crops for melonworm.
For pickleworm, research has suggested that the most reliable sampling method is to begin sampling flower buds (Fig. 7) for small caterpillars before they bore into the fruit (Brewer and Story, 1987). Check with Extension specialists or other growers in your area to find out when pickleworm and melonworm generally appear. In conventional cucurbit production, growers often begin weekly insecticide sprays if larvae are detected in the buds. The same approach may be used with insecticides approved for organic production, although these materials are not generally as effective as conventional insecticides (see section under Management below).
Figure 7. Pickleworm entrance hole in squash blossom. Photo credit: John L. Capinera, University of Florida, Featured Creatures
Because these pests are difficult if not impossible to control once they infest cucurbit plants, and in keeping with the organic approach to pest management, implementation of preventative cultural practices will be the best way to avoid problems associated with pickleworm and melonworm. These include:
- early planting
- sanitation and weed control
- planting less-susceptible varieties
- use of row covers
- trap cropping with squash
If preventive management strategies alone are unable to provide adequate contol, the application of insecticides approved under the National Organic Program (NOP) and/or entomopathogenic nematodes may help to reduce damage. A combination of several strategies will probably be the most effective.
Preventative Management Strategies Early PlantingBefore development of effective insecticides, cucumbers and other susceptible cucurbit crops in the Carolinas were only grown in the early part of the summer, because crops grown after that time were usually destroyed by pickleworm (Anderson and Hofmaster, 1948). Thus organic growers may take advantage of early markets and plant susceptible crops like squash early to avoid serious damage.
Sanitation and Weed ControlAlthough these insect species do not tolerate cold temperatures, immature stages may survive warm winters in the southeastern states by overwintering in plant material. Therefore, removal and destruction of infested plants including vines and fruit following harvest is a good cultural practice to reduce populations (Smith, 1911). Also, some weeds in the cucurbit family, including creeping cucumber (Melothria pendula) and wild balsam apple (Momordica chorantia), may serve as alternate hosts (Elsey et al., 1985). Therefore it is a good practice to remove these plants in areas adjacent to cucurbit crop fields. The USDA–NRCS Plants Database provides additional information on weeds in the cucurbit family, including images and distribution.
Resistant VarietiesResearch has shown that cucurbit resistance to both pickleworm and melonworm is based on oviposition nonpreference (Elsey, 1985). It is likely that there are plant compounds in the less preferred cultivars that deter egg-laying by female moths. A search of the literature did not identify any commercially available cucurbit varieties with resistance to melonworm specified. However, research by Brett et al. (1961) demonstrated marked resistance to pickleworm in the following varieties: Butternut 23, Summer Crookneck, Early Prolific Straightneck, and Early Yellow Summer Crookneck. The varieteis more susceptible to pickleworm are Cozini Zucchini, Black Caserta Zucchini, and Benning's Green Tint Scallop squash.
Row CoversThe use of floating row covers has been shown to exclude pickleworm, melonworm, and other insect pests such as whiteflies and aphids from squash plants (Webb and Linda, 1992). Row covers prevent moths from laying eggs on the plants, and should be applied immediately after planting; however they must be removed to allow for pollination by bees and other pollinating insects. There are two approaches to this depending on the size of the planting and effort one wishes to devote to manipulating row covers:
- Remove row covers permanently after plants begin to flower. This would prevent early-season infestation (probably most important for melonworm) but would of course allow any adult moths present to lay eggs on plants and could result in subsequent damage by larvae.
- After plants begin to flower, remove row covers during the day to allow for pollination, and replace covers in the late afternoon to prevent egg-laying by the nocturnal moths.
Several researchers including Smith (1911) have shown that, because squash is a preferred host for pickleworm, it can be used as a trap crop to deter pickleworm from attacking other cucurbit crops like cantaloupe and cucumber. Smith recommended that squash blossoms be destroyed periodically to keep pickleworms from moving to adjacent cantaloupes. Research in Alabama demonstrated that 6-row cucumber plots (Vlas-Pic variety) bordered on both sides by two rows of squash (Dixie variety) sustained significantly lower pickleworm damage compared with cucumber grown without border rows of squash (Zehnder, unpublished data). However, in these experiments a synthetic insecticide was applied to the trap crop as soon as larvae were detected on plants, which would not be allowed in certified organic production. Organic growers may use approved materials such as spinosad (see below). Squash may also be used as a sentinel crop to detect the first appearance of pickleworms in flowers. This information can be used to help time applications of Bacillus thuringiensis and nematodes, as described below.
Figure 8. Two row perimeter trap crop of Buttercup squash around a main crop of Butternut squash (for cucumber beetle control). Photo credit: Andrew Cavanagh and Ruth Hazzard, University of Massachusetts Extension Vegetable program.
Capinera (2005) reported that, although pickleworm has many natural enemies including predatory beetles, fire ants, and several species of parasitic wasps, none can reliably suppress damage. Other authors suggest that natural enemies have a significant impact on pickleworm and melonworm and that hard insecticides should be avoided to preserve the effectiveness of beneficial organisms (McCleod, 2008). Elsey (1980) reported parasitism of pickleworm eggs by Trichogramma wasps as high as 69%, but this occurred only late in the season. He also reported that fire ants were an important predator of pickleworm pupae.
Application of Entomopathogenic Nematodes and NOP-Approved InsecticidesImportant: Before using any pest control product in your organic farming system:
- Read the label to be sure that the product is labeled for the crop and pest you intend to control,
- Read and understand the safety precautions and application restrictions, and
- Make sure that the brand name product is listed in your Organic System Plan and approved by your USDA-approved certifier. If you are trying to deal with an unanticipated pest problem, get approval from your certifier before using a product that is not listed in your plan—doing otherwise may put your certification at risk.
Note that, although OMRI and WSDA lists are good places to identify potentially useful products, all products that you use must be approved by your certifier. For more information on how to determine whether a pest control product can be used on your farm, see the related article, Can I Use This Input On My Organic Farm?
NematodesNematodes that attack insects are called entomopathogenic nematodes and several species are available commercially for control of various insect pests, particularly those that live in the soil. This is because nematodes survive better in the soil than above ground where they will die when exposed to sunlight and to hot, dry conditions. Several researchers have reported significant control of pickleworm by application of entomopathogenic nematodes such as Steinernema carpocapsae. Shannag et al. (1994) reported that this nematode could effectively reduce pickleworm injury in squash because it has large, enclosed flowers. Because the nematodes are protected in that moist environment they can attack pickleworm larvae before they bore into the fruit. They suggest that nematodes may not be effective on cucurbits with small, open flowers such as cucumber because the nematodes would not be as protected and would desiccate and die from exposure to sunlight. However, Zehnder (unpublished data) found that spray application of Steinernema carpocapsae (strain 25; Biosys Corp.) to cucumber every 4 days beginning when first flower buds were formed significantly reduced pickleworm damage compared to an untreated control.
Bacillus thuringiensisBacillus thuringiensis products, commonly called Bt, contain a toxin produced by the Bt bacteria that will kill certain insects. The Bt variety called “kurstaki” is most effective on caterpillars like pickleworm and melonworm. The Bt toxin must be ingested by the insects for mortality to occur; therefore good spray coverage is essential. There are several OMRI-approved Bt kurstaki products including the following brand names: Agree (Certis), Dipel (Valent), Javelin (Certis), XenTari (Valent). As mentioned above, because the Bt product must be ingested, control of pickleworm may be problematic because the larvae are protected inside leaf and/or flower buds or in fruit. Therefore if pickleworm is present, in addition to good spray coverage, it is important to begin application of Bt when the first buds or open flowers are present, or at least when larvae are first detected in plants. Zehnder (unpublished data) found that spray application of Javelin WG (1.0 lb/acre rate) beginning either with first presence of buds or flowers, or when larvae were first detected, and applied every 4–7 days significantly reduced pickleworm damage to cucumber fruit compared to an untreated control.
Other NOP-Approved InsecticidesTwo additional insecticides approved for organic production and control of melonworm and pickleworm include neem and spinosad. Neem products (e.g., Neemix 4.5™) are botanical insecticides with the active ingredient azadirachtin that is extracted from the neem tree. Neem insecticides are effective against a variety of insect pests through contact toxicity, disruption of insect molting, and feeding deterrence. Labels direct users not to apply neem when honeybees are actively visiting flowers in the area.
The active ingredient in spinosad (e.g., Entrust™) insecticide is derived by microbial fermentation and is effective against a wide variety of insect pests. Spinosad is safe to most beneficial insects; however the wet spray can kill bees. The label indicates that once the spray dries there is no risk to bees foraging on plants.
References and CitationsAnderson, R., and R. Hofmaster. 1948. Control of pickleworms on cucumber and cantaloupe. Journal of Economic Entomology 41: 334–335. (Available online at: http://jee.oxfordjournals.org/content/41/2/334) (verified 20 Sept 2011).
Brett, C., C. McCombs, and D. Daugherty. 1961. Resistance of squash varieties to the pickleworm and the value of resistance to insecticidal control. Journal of Economic Entomology 54: 1191–1197. (Available online at: http://jee.oxfordjournals.org/content/54/6/1191) (verified 20 Sept 2011).
Brewer, M. J., and R. N. Story. 1987. Larval spatial patterns and sequential sampling plan for pickleworm, Diaphania nitidalis (Stoll) (Lepidoptera: Pyralidae), on summer squash. Environmental Entomology 16: 539–544. (Available online at: https://doi.org/10.1093/ee/16.2.539) (verified 12 Jun 2019).
Capinera, J. 2005. Melonworm, Diaphania hyalinata Linnaeus (Insecta: Lepidoptera: Pyralidae) [Online]. Publication # EENY-163. Entomology and Nematology Department, Florida Cooperative Extension Service, Institute of Food and Agricultural Sciences, University of Florida. (Available online at: http://edis.ifas.ufl.edu/in320) (verified 20 Sept 2011).
Capinera, J. 2005. Pickleworm, Diaphania nitidalis (Stoll) (Insecta: Lepidoptera: Pyralidae) [Online]. Publication # EENY-164. Entomology and Nematology Department, Florida Cooperative Extension Service, Institute of Food and Agricultural Sciences, University of Florida. (Available online at: http://edis.ifas.ufl.edu/in321) (verified 29 July 2011).
Elsey, K. D. 1980. Pickleworm: Mortality on cucumbers in the field. Environmental Entomology 9: 806–809. (Available online at: http://ee.oxfordjournals.org/content/9/6/806) (verified 20 Sept 2011).
Elsey, K. D., J. E. Pena, and V. H. Waddill. 1985. Suitability of potential wild hosts of Diaphania species in southern Florida. The Florida Entomologist 68: 682-686. (Available online at: http://www.jstor.org/stable/3494874) (verified 20 Sept 2011).
Elsey, K. D. 1985. Resistance mechanisms in Cucurbita moshata to pickleworm and melonworm. Journal of Economic Entomology 78: 1048–1051. (Available online at: http://dx.doi.org/10.1093/jee/78.5.1048) (verified 20 Sept 2011).
McCleod, P. 2008. Identification, biology and management of insects attacking vegetables in Arkansas. (Available online at: http://comp.uark.edu/~pjmcleod/arkveginsects/) (verified 29 July 2011).
Shannag, H., S. Webb, and J. Capinera. 1994. Entomopathogenic nematode effect on pickleworm under laboratory and field conditions. Journal of Economic Entomology 87: 1205–1212. (Available online at: http://dx.doi.org/10.1093/jee/87.5.1205) (verified 20 Sept 2011).
Smith, R. I. 1911. Two important cantaloupe pests. North Carolina Agricultural Experiment Station Bulletin 214: 101–146. (Available online at: ) (verified 20 Sept 2011).
Webb, S., and S. Linda. 1992. Evaluation of spunbonded polyethylene row covers as a method of excluding insects and viruses affecting fall-grown squash in Florida. Journal of Economic Entomology 85: 2344–2352. (Available online at: http://jee.oxfordjournals.org/content/85/6/2344) (verified 20 Sept 2011).
Webb, S., and J. Capinera. 1995. Management of pickleworm with entomopathogenic nematodes. Proceedings of the Florida State Horticultural Society 108: 242–245. (Available online at: http://www.fshs.org/Proceedings/Password%20Protected/1995%20Vol.%20108/242-245%20%28WEBB%29.pdf) (verified 29 July 2011).
Webb, S. E. 2010. Insect management for cucurbits [Online]. Publication # ENY-460. Entomology & Nematology Department, Florida Cooperative Extension Service, Institute of Food and Agricultural Sciences, University of Florida. (Available online at: http://edis.ifas.ufl.edu/in168) (verfied 29 July 2011).
Zehnder, G. unpublished data. Entomopathogenic nematodes, Bacillus thuringiensis, and a squash trap crop reduce pickleworm damage in cucumber (unpublished; data available upon request; zehnder@clemson.edu).
Additional ResourcesSorensen, K. A., and J. R. Baker (ed.). 2002. Pickleworm and Melonworm. Insect and related pests of vegetables. Center for Integrated Pest Management. North Carolina State University. Available online at: http://ipm.ncsu.edu/ag295/html/pickleworm_melonworm.htm (verified 29 July 2011).
This is an eOrganic article and was reviewed for compliance with National Organic Program regulations by members of the eOrganic community. Always check with your organic certification agency before adopting new practices or using new materials. For more information, refer to eOrganic's articles on organic certification.
eOrganic 5320
Video Clips about Organic Farming by eOrganic
- Videos about Organic Dairy Farming - Produced by University of Vermont and the eOrganic Dairy Team
- Menalled, F. et al. 2015. Video: Using Sheep to Terminate Cover Crops in Organic Farming. Montana State University video. Available at http://articles.extension.org/pages/73046
- Anderson, J. and K. Bielek. 2013. Video: A whole farm approach to incorporating pasture raised organic poultry and a novel cereal grain (Naked Oats) into a multi-year organic rotation. eOrganic video. Available at http://articles.extension.org/pages/70084
- Anderson, J. and K. Bielek. 2016. Incorporating Pasture-Raised Organic Poultry and Naked Oats into an Organic Rotation. eOrganic video. Available at http://articles.extension.org/pages/73680
- Topdressing Organic Hard Winter Wheat to Enhance Grain Protein - University of Maine
- Dyck, E. F. Kutka, S. Zwinger. 2017. Growing and Dehulling the Ancient Grains Einkorn, Emmer and Spelt. eOrganic video. Available at https://articles.extension.org/pages/74475
- Kolb, L. 2013. Weed Control in Organic Spring Cereals. Available at https://articles.extension.org/pages/68312
- Morrison, R. and C. Matthews. 2016. CSI: Brown Marmorated Stink Bug (BMSB) Egg Mass Damage. eOrganic video. Available at http://articles.extension.org/pages/73495
- Shennan, C. J. Muramoto, D. Nieto, M. Zavatta. 2016. CalCORE Research: Improving Biological Control of Lygus Bug and Cabbage Aphid. eOrganic video. Available at https://articles.extension.org/pages/73916
- Thomsen, E. 2018. Simple Soil Tests 2: Soil Biological Tests: Solvita Soil Test. eOrganic video. Available at https://www.youtube.com/watch?v=KRlvG_isfW8
- Thomsen, E. 2018. Simple Soil Tests Part 1: Sieve and Bucket Test, Hose Test. eOrganic video. Available at https://www.youtube.com/watch?v=meFfOpNb75c
- Vegetable Farmers and their Weed Control Machines - University of Vermont Center for Sustainable Agriculture
- Vegetable Farmers and their Sustainable Tillage Practices - University of Vermont Center for Sustainable Agriculture
- Vegetable Farmers and their Innovative Cover Cropping Techniques - University of Vermont Center for Sustainable Agriculture
- Farmers and their Diversified Horticultural Marketing Strategies - University of Vermont Center for Sustainable Agriculture
- Weed 'Em and Reap Part 1. Tools for Organic Weed Management in Vegetable Cropping Systems - Oregon State University Department of Horticulture
- Weed 'Em and Reap Part 2. Reduced Tillage Strategies for Vegetable Cropping Systems - Oregon State University Department of Horticulture
- Organic Vegetable Production - University of Florida IFAS Extension Virtual Field Day
- Identifying and Scouting for Late Blight on Organic Farms - New York State Integrated Pest Management Program, Cornell University
- Blubaugh, C. 2017. Scouting Vegetable Crops: An Introduction for Farmers. eOrganic video. Available at https://articles.extension.org/pages/74490
- Blubaugh, C., W. Snyder and J. Reganold. 2017. Identifying Syrphid Fly Larvae: Important Beneficial Insects in Controlling Aphids. eOrganic video. Available at https://articles.extension.org/pages/74501
- Brennan, E. 2015. A Biological Control Buffet in the Salad Bowl of America. Available at http://articles.extension.org/pages/73410
- Brennan, E. 2015. Efficient Intercropping for Biological Control of Aphids in Transplanted Organic Lettuce. Available at http://articles.extension.org/pages/72642
- DuPont, T. 2012. Pest Management Case Study. Quiet Creek Farm, Kutztown, PA. Penn State Extension Start Farming Video. Available at https://articles.extension.org/pages/64622
- DuPont, T. 2012. Plant Propagation Case Study. Quiet Creek Farm, Kutztown, PA. Penn State Extension Start Farming Video. Available at https://articles.extension.org/pages/64623
- Grauer, J., M. Manning, L. Wyatt. 2014. Addressing Critical Management Challenges in Organic Cucurbit Production. eOrganic video. Available at http://articles.extension.org/pages/71052
- Moncada, K. 2017. Video Series on Soybean and Dry Bean Research at the University of Minnesota. Available at https://articles.extension.org/pages/74285
- Shennan, C. et al. 2016. CalCORE: Connecting California Farmers and Scientists to Improve Rotational Strawberry and Vegetable Systems. eOrganic video. Available at http://articles.extension.org/pages/73626
- Shennan, C. J. Muramoto, D. Nieto, M. Zavatta. 2016. CalCORE Research: Controlling Soilborne Diseases in California’s Strawberry Industry with Anaerobic Soil Disinfestation (ASD). eOrganic video. Available at https://articles.extension.org/pages/73897
This is an eOrganic article and was reviewed for compliance with National Organic Program regulations by members of the eOrganic community. Always check with your organic certification agency before adopting new practices or using new materials. For more information, refer to eOrganic's articles on organic certification.
eOrganic 3549
Video: Using Sheep to Terminate Cover Crops in Organic Farming
This video describes ongoing interdisciplinary research that explores how the use of domestic sheep—rather than traditional farming equipment—to manage fallow land and terminate cover crops may enable farmers who grow organic crops to save money, reduce tillage, manage weeds and pests, and reduce the risk of soil erosion. Researchers from Montana State University and North Dakota State University are working on a USDA-National Institute of Food and Agriculture (NIFA) Organic Transitions project: Reducing Tillage in Organic Crop Systems: Ecological and Economic Impacts of Targeted Sheep Grazing on Cover Crops and Weed management, Soil Health and Stability, Carbon Sequestration and Greenhouse Gas Emissions. Additional information about the project is available on the Montana State University website here.
Researchers: Fabian Menalled, Patrick Hatfield, Perry Miller, Anton Bekkerman, Devon Ragen
Camera: Steve Spence, Casey Kanode, Jared Berent
Editing: Case Kanode, Steve Spence
Recording available on YouTube at https://www.youtube.com/watch?v=Y5w25UgWMTs
This is an eOrganic article and was reviewed for compliance with National Organic Program regulations by members of the eOrganic community. Always check with your organic certification agency before adopting new practices or using new materials. For more information, refer to eOrganic's articles on organic certification.
eOrganic 13042
Food Hub Feasibility in Oregon’s Mid-Willamette Valley: Interviews with Conventional and Organic Small and Mid-Sized Farmers
eOrganic authors:
Eliza Smith, Oregon State University
Javier Fernandez-Salvador, Oregon State University
A food hub is a centralized location, either brick and mortar or online-based, that connects farmers and food buyers. Food hubs are becoming an increasingly common model for producers to sell their products in local markets (Colasanti et al., 2018). Food hubs may be a website where farmers post their products for sale, or a physical location that provides services to growers such as aggregation and distribution management, a commercial kitchen, or a USDA meat-processing facility (Colasanti et al., 2018). Food hubs may also be a vehicle to educate the community on the value of buying products from local producers (Cantrell and Heuer, 2014). Food hubs can be especially beneficial to small growers because they provide infrastructure and services that those growers may not have the means to provide on their farms (Conner et al., 2017). In fact, the 2017 Food Hub Survey showed that over 90% of food hubs had “increasing small and mid-sized producers’ access to markets” included in their mission (Colasanti et al., 2018). A previous study showed that food hubs are most successful in metropolitan areas because of their accessibility to growers, wholesale buyers, and retail consumers (Fischer et al., 2013). For example, in Portland, Oregon the nonprofit Ecotrust organizes a physical location food hub, The Redd on Salmon Street.
Introduction to the Food Hub Feasibility SurveySalem is the capital of Oregon with a population of 168,000. It is the second-largest city in Oregon, behind Portland. In 2016, the City of Salem proposed a food hub as one option to revitalize a low-income area that was slated for improvement with urban renewal dollars. The city collaborated with ECONorthwest consultants and the local OSU Extension Service Small Farms Program to develop, conduct, analyze, and present the results of a survey of small and mid-sized farmers (both organic and conventional) in the Salem area to assess their interest in a potential food hub.
The survey consisted of two sections with both multiple-choice and open-ended questions. The first section included general questions about the farming operation (location, acreage, what products they sell, when the products are available for sale, and where they currently sell their products). The second section was designed to assess the farmers' interest in a food hub in Salem, and included questions about farmers' interest in increasing local sales, whether they thought a food hub was needed in the Mid-Willamette Valley, and their top farming challenges. The second section also asked what services a food hub could provide that would benefit them, as well as whether they would be interested in participating in a food hub, where they would ideally like it to be located, and barriers they see to their participation in a food hub. Since this survey was only an initial inquiry to see whether the farmers were interested in a food hub, more specific questions like what farmers would pay for services provided by the food hub were not included.
A total of 19 small and mid-sized farmers (10.5% of the estimated total in the region) were interviewed for the survey, from September 2016 to March 2017; 18 on-site at their farms and one over the phone. While the survey was being developed, a database of small farmers in Oregon's Mid-Willamette Valley was compiled from internet searches, extension contact lists, and county tax assessor data. Survey participants were contacted from this database. As in most research that includes interviews or surveys, the data was limited to responses from farmers that chose to participate. Many of the farmers who responded to the request for an interview had been previously involved with the OSU Extension Service (participated in research, answered surveys, hosted workshops, etc.). A breakdown of what crops/products the survey participants grew or produced is shown in Table 1.
Table 1. Number and percentage of participants that farm and sell various products Sales outlet On-farm Farmers' Market CSA Restaurant Grocery (retail) Institutions Wholesale Online Other Number of participants that sell via that outlet 7 5 7 6 4 0 8 0 2Seven of the farmers interviewed were certified organic and the remaining 12 were not. The non-organic farmers were a mix of six conventional farms and six farms that were practicing alternative agriculture (no-spray, ecological, etc.) but were not certified organic.
The same interviewer conducted the interviews with producers for consistency of data collection. Data were analyzed in one and two-way tables by breaking the participants into sub-groups based on farm location, years of farming experience, farmed acreage, products farmed and sold, and organic certification status.
Key Findings from the Survey- Three-quarters of the farmer participants had heard the term food hub before the survey. It was important to ensure that all of the survey participants had a common understanding of a food hub, as that would affect their responses.
- Participants sold their products through a variety of outlets, ranging from farmers' markets to wholesale (Table 2).
Table 2. Sales outlets that participants use to sell their products
Service the food hub could provide Responded that it would be helpful Community Education 84% Value added processing 74% Aggregation 68% Direct Sales 68%- All but one of the farmers interviewed for the survey were interested in participating in a food hub in Salem, Oregon. The producer that was not interested in participating had a variety of long term wholesale buyers and was not looking to expand farm sales.
- Community education was the most common option chosen by all participants interested in a food hub when asked what services they would like a food hub to provide (Table 3). Value-added processing, direct sales, distribution, and aggregation were of secondary interest to participants (Table 3).
Table 3. Services that farmers said would be helpful as part of a food hub, ranked from highest to lowest percentage of respondents.
Service the food hub could provide Responded that it would be helpful Community Education 84% Value added processing 74% Aggregation 68% Direct Sales 68% Distribution 63% Transportation 58% Cold Storage 58% Marketing Support 58% Local label 53% GAP/food safety cert. assistance 47% USDA meat processing facility 42% Organic certification assistant 37% Freezer storage 26% Light processing 26% Dry product handling 21%- All of the certified organic farmers grew mixed vegetables, which shaped many of their responses from product availability to services they want the food hub to provide. Only 58% of the non-organic farmers grew mixed vegetables.
- All of the meat producers surveyed said processing is their primary farming challenge because of the limited number of USDA meat-processing facilities in the region that will work with small producers. All seven meat producers surveyed said a USDA meat-processing facility was their top priority for a service the food hub could provide. Two of the seven meat producers surveyed were certified organic. For their meats to be sold as organic after processing, the facility would have to be certified organic in addition to being USDA-certified.
- Three main concerns that emerged from the interviews with both organic and non-organic farmers about participating in the food hub were: 1) they would need to set their prices too low, 2) lack of consumer demand for their products in the geographical area around the food hub, and 3) too much competition from larger farms who would also participate in a food hub.
- Both organic and non-organic farmers were concerned that end-consumers and/or buyers would not go to the food hub to buy their products. Considering that, they requested that the food hub provide community education about the value of buying local agricultural products.
- The participant with one of the largest farms in the survey (100+ acres) that has robust transportation and wholesale distribution systems for their organic produce, expressed concern that the transportation limitations of smaller farmers due to fewer vehicles and employees (regardless of certification status) would limit large-volume accounts at the food hub.
- Large-volume buyers (wholesale) commonly have an interest in purchasing goods at rates lower than direct-to-consumer prices. This decrease in profit margin would not prove sustainable or enticing for small and mid-sized farm operations, causing many of the survey participants to doubt or question the implementation of a similar business model, as reported in the ECONorthwest writeup of the survey results for the City of Salem (ECONorthwest, 2017).
It is important to compare the responses from these two groups of participants because organic and non-organic products command different prices, which we expected may affect the farmers' responses.
- On average, the organic growers had been farming more years than the non-organic farmers surveyed.
- A much higher percentage of organic farmers did not think a food hub was necessary in the Mid-Willamette Valley (29%) as compared to non-organic farmers (8%). Many of the organic farmers said that they would gladly participate in a food hub, but were worried that there wouldn't be enough people or companies in Salem to buy their products. A lot of the organic farmers take their products to Portland, the largest metropolitan area in the state, to sell them where the demand for organic foods is more mainstream and verified by existing organic sales in the city. Producers surveyed were concerned that it would be more difficult to sell their organic products in Salem and get the price they need for them.
- The report presented by ECONorthwest stressed that overall, consumer energy for the local foods movement is not as strong in Salem as in other parts of the Mid-Willamette Valley (2017). This demonstrates a need for end-consumer education about buying local agricultural products, which was a point that all of the farmers mentioned and considered important.
- Our study supported this finding, as many survey participants cited a potential lack of demand for locally produced foods by consumers as a factor that could contribute towards hesitation about building a food hub.
Food hubs may be useful and important resources for farmers looking for new local outlets to sell their products. This survey indicated a great interest from small and mid-sized farmers in participating in a food hub. It also collected a variety of concerns from the farmers about price point, consumer education, and competition from larger farms. As backed by other studies and farmer responses, we determined that for a food hub to be successful, farmers' interest in participating must be met by sufficient consumer demand for local agricultural products, and this may be particularly true for organic products. Establishing a food hub is a large undertaking and conducting preliminary surveys to gauge interest from both farmers and consumers is an important first step before investing in more comprehensive assessments. When farmers are considering participation in a food hub, it is important to ask many of the same questions that a survey like this would pose, such as: What services will the food hub provide? What are your price requirements to sell at a food hub? Is the food hub in a convenient location? And, do you see any potential barriers to your participation in a food hub?
Additional Resources- National Good Food Network Food Hub Center (The Wallace Center). Provides a variety of resources, including: webinars about existing food hubs, a link to the USDA food hub directory, a food hub consultant database, funding sources for food hubs, and research about food hubs.
- USDA Regional Food Hub Resource Guide. Summary of what a food hub is, its impacts, economic viability, potential barriers to growth and possible solutions to those barriers. Starting on page 29, there are listings of various funding sources available to help establish a food hub.
- USDA Agricultural Marketing Service. General information about food hubs and links to resources. The USDA AMS Food Hub Map is a visual representation of the USDA food hub directory.
- A Food Hub Facility Design Case Study. A case study of the establishment of the Tuscarora Organic Growers food hub in Pennsylvania, focusing on the physical building of the food hub. Includes building dimensions and operational expenses.
- Healthy Food Access Portal: Food Hubs. This webpage provides an overview of food hubs, including resources, strategies, challenges and food hub success stories from all over the U.S.
- Barham, J., D. Tropp, K. Enterline, J. Farbman, J. Fisk, and S. Kiraly. 2012. Regional food hub resource guide [Online]. USDA Agricultural Marketing Service. Available at: http://dx.doi.org/10.9752/MS046.04-2012 (verified 25 Sep 2018)
- Barham, J., and F. Delgado. 2015. Building a Food Hub From the Ground Up: A Facility Design Case Study of Tuscarora Organic Growers [Online]. USDA Agricultural Marketing Service. Available at: https://www.ams.usda.gov/publications/content/building-food-hub-ground-facility-design-case-study-tuscarora-organic-growers (verified 5 Mar 2018)
- Cantrell, P., and B. Heuer. 2014. Food hubs: Solving local. Small-farm aggregators scale up with larger buyers [Online]. The Wallace Center at Winrock International. Available at: http://www.ngfn.org/resources/ngfn-database/knowledge/Food%20Hubs%20-%20Solving%20Local.pdf (verified 5 Mar 2018)
- Colasanti, K., J. Hardy, J. Farbman, R. Pirog, J. Fisk, and M. W. Hamm. 2018. Findings of the 2017 national food hub survey [Online]. Michigan State University Center for Regional Food Systems & The Wallace Center at Winrock International. Available at: www.foodsystems.msu.edu/2017foodhubsurvey (verified 25 Sep 2018)
- Conner, D. S., K. Sims, R. Berkfield, and H. Harrington. 2017. Do farmers and other suppliers benefit from sales to food hubs? Evidence from Vermont. Journal of Hunger & Environmental Nutrition, 1–10. Available online at: http://doi.org/10.1080/19320248.2017.1378602 (verified 25 Sep 2018)
- ECONorthwest. 2017. Salem community food study [Online]. Available at: https://www.cityofsalem.net/CityDocuments/salem-community-food-study-full-with-appendix.pdf (verified 5 Mar 2018)
- Ecotrust. 2018. The Redd on Salmon Street [Online]. Available at: https://ecotrust.org/project/the-redd-on-salmon-street/ (verified 5 Mar 2018)
- Fischer, M., M. Hamm, C. S. Mott, R. Pirog, J. Fisk, J. Farbman, and S. Kiraly. 2013. Findings of the 2013 national food hub survey [Online]. Michigan State University Center for Regional Food Systems & The Wallace Center at Winrock International. Available at: http://www.canr.msu.edu/foodsystems/uploads/files/2013-food-hub-survey.pdf (verified 5 Mar 2018)
- Healthy Food Access Portal. 2018. Food Hubs [Online]. Robert Wood Johnson Foundation Center for Healthy Food Access. Available at: http://www.healthyfoodaccess.org/launch-a-business-models-food-hubs (verified 5 Mar 2018)
- Hellwinckel, C., and M. Velandia. 2016. Knoxville regional food hub feasibility study [Online]. University of Tennessee Institute of Agriculture. Available at: https://knoxmpc.org/news/2016/0324/Knoxville%20Regional%20Food%20Hub%20Feasibility%20Study_FINAL_2016.pdf (verified 5 Mar 2018)
- National Good Food Network. 2009. Food Hub Center [Online]. Winrock International. Available at: http://www.ngfn.org/resources/food-hubs (verified 5 Mar 2018)
- USDA Agricultural Marketing Service. 2018. Food Value Chains and Food Hubs [Online]. Available at: https://www.ams.usda.gov/services/local-regional/food-hubs (verified 5 Mar 2018)
- USDA Aricultural Marketing Service. 2018. 206 Food Hubs [Online]. Available at: https://search.ams.usda.gov/foodhubs/googleMapFull.aspx (verified 5 Mar 2018)
This is an eOrganic article and was reviewed for compliance with National Organic Program regulations by members of the eOrganic community. Always check with your organic certification agency before adopting new practices or using new materials. For more information, refer to eOrganic's articles on organic certification.
eOrganic 25213
Fire Blight Control for Organic Orchards: Moving Beyond Antibiotics
eOrganic author:
David Granatstein, Washington State University
Fire blight is a serious disease of apple and pear caused by the bacterium Erwinia amylovora. It originated in the United States and is now found in many parts of the world. Most domesticated apple and pear cultivars have some degree of susceptibility to infection. Damage is not cosmetic, but reduces crop yield and may kill entire trees. Prior to implementation of the National Organic Program (NOP), a number of U.S. certifiers allowed the use of the antibiotics oxytetracycline and streptomycin for control of this disease, as they are naturally occurring molecules produced by soil microorganisms. However, the NOP classified them as synthetics but allowed their use for fire blight control only. The National Organic Standards Board has voted to remove these antibiotics from the national list of allowed materials, and their use will be prohibited after October 2014. Due to the potentially devastating damage from this disease, organic apple and pear growers are looking for viable non-antibiotic control measures.
Breeding for ResistanceThe ideal solution to fire blight is genetic resistance bred into both the scion (fruit-bearing portion) and rootstock of the tree. The ‘Geneva’ series apple rootstocks do exhibit a high level of resistance to fire blight, but do not confer resistance to the scion grafted onto them. No highly resistant scion cultivars have been identified that also have the requisite fruit quality characteristics needed for commercialization. Several cultivars of pear developed by the USDA and Agriculture and Agri-Food Canada do exhibit increased resistance. Breeding programs are looking at sources of resistance in other wild apple species that have better fruit quality potential, and progress can be expected over the next 10-15 years.
Biological and Chemical ControlApplication of antibiotics has been the primary practice used to manage fire blight for more than 50 years. Antibiotics are effective and fast-acting, and can be used in concert with disease prediction models (e.g., COUGARBLIGHT, MARYBLYT) so treatments may only be made when risk of infection is high. Research on biological control practices has been conducted since the 1980s, and several products have been commercialized such as Blight Ban®A506 (Pseudomonas fluorescens strain A506). However, until recently, no products exhibited efficacy similar to antibiotics. In 2012, the yeast product Blossom Protect™ (Aureobasidium pullulans) debuted in the U.S. market and has performed well for the past two seasons. Other materials such as Serenade® MAX (Bacillus subtilis), Double Nickel 55™ (Bacillus amyloliquefaciens), and soluble copper (e.g., Cueva®) are also available and organic-compliant, providing growers with several options to combine into an integrated fire blight management program. In addition, lime sulfur, commonly used by apple growers as a blossom thinner to reduce crop load, has been shown to exert control of fire blight when applied during bloom.
IMPORTANT: Before using any pest control product in your organic farming system:
- Read the label to be sure that the product is labeled for the crop and pest you intend to control, and make sure it is legal to use in the state, county, or other location where it will be applied
-
Read and understand the safety precautions and application restrictions
-
Make sure that the brand name product is listed in your Organic System Plan and approved by your USDA-approved certifier. If you are trying to deal with an unanticipated pest problem, get approval from your certifier before using a product that is not listed in your plan—doing otherwise may put your certification at risk.
Note that OMRI and WSDA lists are good places to identify potentially useful products, but all products that you use must be approved by your certifier. For more information on how to determine whether a pest control product can be used on your farm, see the article, Can I Use This Input On My Organic Farm?
Time to Test AlternativesOrganic growers exporting fruit to the European Union were prohibited from using antibiotics, and they tested various approaches and products that were successful in certain locations and with certain cultivars. More recently, an organic fire blight control project for Oregon, Washington, and California, led by Dr. Ken Johnson of Oregon State University, has made significant progress in testing many of these materials and evaluating their efficacy as well as potential combinations and timings for best results. Growers need to test alternative controls on their own sites with their specific cultivars to prepare for the loss of antibiotics.
The following resources are available to help organic growers learn more about fire blight control alternatives. These include eOrganic webinars by Dr. Johnson, a new publication Grower Lessons and Emerging Research for Developing an Integrated Non-Antibiotic Fire Blight Control Program in Organic Fruit from The Organic Center, a recent journal article (Johnson and Temple, 2013) Evaluation of strategies for fire blight control in organic pome fruit without antibiotics), and an annotated powerpoint by Dr. Johnson summarizing the research progress to date. The applicability of the information presented in these various sources will undoubtedly vary by region, crop, orchard age, training system, and cultivar, so growers should be conducting some simple field evaluations of their own while the research proceeds.
One issue not fully resolved is that of fruit marking or russetting—a cosmetic defect on the skin of fruit caused by phytotoxicity of control materials when applied at a susceptible fruit stage. If a material provides a high level of fire blight control but russets fruit and renders it unmarketable in commercial channels, then it will not be an acceptable material for most growers.
ConclusionSignificant progress has been made in the past several years on non-antibiotic fire blight control methods that would be compliant on organic orchards. Well-vetted recommendations are not yet available, and thus growers need to be testing these new materials and ideas on their own orchards in the meantime. Ultimately, genetic resistance to the disease will provide the most sustainable alternative but this is likely decades away. However, growers can test small plantings of some of the reputedly more resistant cultivars now, observe their resistance, fruit quality, and horticultural needs, and develop their own markets for those new cultivars. The demand for organic apples and pears continues to increase, and growers need well-proven fire blight control approaches to allow them to respond to this demand while minimizing risks from the disease.
References and Citations Additional Resources- Granatstein, D., T. Smith, and G. Peck. 2011. The role of tree genetics in controlling fire blight in apples and pears. Organic tree fruit industry work group paper. (Available online at: http://www.tfrec.wsu.edu/pdfs/P2396.pdf) (verified 3 March 2014)
- Johnson, K. 2014. Non-antibiotic control of fire blight for organic orchards. Annotated presentation from Wilbur-Ellis organic grower meeting, Benton City, WA, Jan. 23, 2014. (Available online at: http://www.tfrec.wsu.edu/pdfs/P2850.pdf) (verified 3 March 2014)
- Johnson, K. B., R. Elkins, and T. Smith. 2013. Research update on non-antibiotic control of fire blight. eOrganic webinar, Oct. 15, 2013. (Available online at: http://www.extension.org/pages/67392/research-update-on-non-antibiotic-control-of-fire-blight-webinar#.UxUjYs7ag21) (verified 3 March 2014)
- Johnson, K. B. and Temple, T. 2013. Evaluation of strategies for fire blight control in organic pome fruit without antibiotics. Plant Disease 97:402–409. (Available online at: http://apsjournals.apsnet.org/doi/pdf/10.1094/PDIS-07-12-0638-RE) (verified 3 March 2014)
- Johnson, K. B. 2005. Fire blight of apple and pear. The Plant Health Instructor. DOI: 10.1094/PHI-I-2000-0726-0. Basic description of the disease, its symptoms, life cycle, and management. (Available online at: http://www.apsnet.org/edcenter/intropp/lessons/prokaryotes/Pages/FireBlight.aspx) (verified 3 March 2014)
- National Organic Standards Board. 2013. Petition to remove the expiration date for tetracycline on 205.601. National Organic Program, USDA-AMS, Washington, DC. 44 pp. (Available online at: http://www.ams.usda.gov/AMSv1.0/getfile?dDocName=STELPRDC5103808) (verified 3 March 2014)
- Norelli, J., K. Evans, and M. Wisniewski. 2012. Identifying fire blight resistance in M. sieversii for scion breeding. Final report, Washington Tree Fruit Research Commission.(Available online at: http://jenny.tfrec.wsu.edu/wtfrc/PDFfinalReports/2012FinalReports/NorelliFireBlightFinal.pdf) (verified 3 March 2014)
- Ostenson, H., and D. Granatstein. 2013. Grower lessons and emerging research for developing an integrated non-antibiotic fire blight control program in organic fruit. Critical issue report, The Organic Center, Washington, DC. (Available online at: http://organic-center.org/wp-content/uploads/2013/07/TOC_Report_Blight_2b.pdf) (verified 3 March 2014)
- Smith, T. 2010. The CougarBlight 2010 fire blight risk model. Washington State University Extension, Wenatchee, WA. (Available online at: https://extension.wsu.edu/chelan-douglas/agriculture/treefruit/pestmanagement/cb2010fireblightriskmodel/) (verified 4 Jun 2019)
- Steiner, P. W., T. van der Zwet, and A. R. Biggs. 2013. Fire blight of apple. (Available online at: http://www.extension.org/pages/60354/fire-blight-of-apple#.UxUYdM7ag21) (verified 3 March 2014)
- Granatstein, D. 2014. Fire blight control in organic apples and pears web page. Washington State University Extension, Wenatchee, WA. (Available online at: http://www.tfrec.wsu.edu/pages/organic/fireblight) (verified 3 March 2014)
- Smith, T. 2012. Fire blight management in the Pacific Northwest USA. Washington State University Extension website. (Available online at: http://treefruit.wsu.edu/crop-protection/disease-management/fire-blight/) (verified 4 Jun 2019)
- Sobiczewski, P., M. Kaluzna, and J. Pulawska (eds.). 2011. XII International Workshop on Fire Blight. Acta Horticulturae (ISHS) 896. This is the proceedings of an international fire blight workshop held every three years. (Parts are available to the public online at: http://www.actahort.org/books/896/index.htm) (verified 3 March 2014)
- Turechek, W. W., and A. R. Biggs. 2015. Maryblyt v. 7.1 for Windows: An Improved Fire Blight Forecasting Program for Apples and Pears. Plant Health Progress. (Available at: http://www.doi.org/10.1094/PHP-RS-14-0046 (verified 10 Jun 2019)
This is an eOrganic article and was reviewed for compliance with National Organic Program regulations by members of the eOrganic community. Always check with your organic certification agency before adopting new practices or using new materials. For more information, refer to eOrganic's articles on organic certification.
eOrganic 10624
Current and Future Prospects For Biodegradable Plastic Mulch in Certified Organic Production Systems
eOrganic authors:
Dr. Andrew T. Corbin Ph.D., Washington State University
Dr. Carol A. Miles Ph.D., Washington State University
Jeremy Cowan, Washington State University
Dr. Douglas G. Hayes Ph.D., University of Tennessee
Dr. Jennifer Moore-Kucera Ph.D., Texas Tech University
Dr. Debra A. Inglis Ph.D., Washington State University
Certified organic farmers are currently allowed to use conventional polyethylene mulch, provided it is removed from the field at the end of the growing or harvest season. To some, such use represents a contradiction between the resource conservation goals of sustainable, organic agriculture and the waste generated from the use of polyethylene mulch. One possible solution is to use biodegradable plastic as mulch, which could present an alternative to polyethylene in reducing non-recyclable waste and decreasing the environmental pollution associated with it. This article explains how biodegradable plastic mulches are made; how biodegradability is measured; current techniques on evaluating biodegradable mulches; and research and policy progress to date. The purpose is to inform agricultural professionals, farmers, and policy makers about the suitability of biodegradable plastic mulches for use in certified organic agriculture. A glossary is provided at the end of this publication which includes definitions and terms commonly used when describing biodegradable plastic mulch. Terms included in the glossary are in bold text.
Plastic mulch films in agriculturePolyethylene plastic mulch is widely used for crop production in the U.S. and worldwide, because it controls weeds, conserves soil moisture, increases soil temperature, improves crop yield and quality, has a relatively low cost, and is readily available (Schonbeck and Evanylo, 1998; Corbin et al., 2009; Miles et al., 2012). However, the sustainability of producing crops through the use of polyethylene mulch has been called into question because polyethylene mulch is made of non-renewable, petroleum-based feedstock, is generally only used for one growing season, and cannot be recycled in most regions. Recycling is limited because the mulch may be contaminated with soil and agrochemicals, specialized baling equipment is required before hauling, and facilities for recycling are often a long distance away. (Garthe and Kowal, 1993).
The high volume of waste generated by polyethylene mulches both in the field and in landfills raises many concerns (Figure 1). For example, in 2004 in the U.S., 143,000 tons of plastic mulch were disposed. While much of this waste entered the landfill at a cost to growers of up to $100 per acre, some was burned on site (Shogren and Hochmuth, 2004). Burning of polyethylene mulch can have undesirable environmental impacts, such as the release of dioxins as an airborne pollutant (Levitan, 2005; Lemieux, 1997). While there are no federal regulations restricting the open burning of plastics, the practice is banned in several states or counties in the U.S. (EPA, 2011; OAR, 2013). Utilization of polyethylene mulch by soil microorganisms in landfills is negligible, with the microbial conversion and abiotic oxidation products possibly consisting of environmentally harmful chemicals such as aldehydes and ketones (Hakkarainen and Albertsson, 2004). The use of biodegradable mulches could save significant labor and disposal costs, conserve resources, and decrease pollution (Smith et al., 2008).
Figure 1. (a) Typical post-season polyethylene plastic mulch waste in the field. (b) Ready for transport to the landfill. Photo Credit: C. Miles, Washington State University. From Corbin et al., 2013.
For these reasons, both new and experimental mulches that are designed to biodegrade (mineralize) fully into carbon dioxide and water, have been developed by industry and academic institutes over the past 25 years. To be a viable alternative in organic crop production, biodegradable plastic mulch must perform comparably to polyethylene mulch, especially in regard to durability and the ability to block light to prevent weed germination. Ideally, once the biodegradable mulch's useful service life has ended, it is plowed into the soil where it should degrade. In the short-term, the mulch should lose mechanical strength and undergo a reduction in the degree of polymerization (Figure 2), or depolymerization, thereby making the polymer molecules accessible to microorganisms. Ultimately, the mulch should undergo at least 90% mineralization within a two-year period.
Figure 2. (a) Starch-based biodegradable plastic mulch (BioAgri®) in experimental field plots during harvest, 135 days after laying mulch. (b) 9 months post-harvest on soil surface, 348 days after laying mulch. (c) 9 months post-incorporation, 348 days after laying mulch. Photo Credits: J. Cowan (2a) and C. Miles (2a, 2b), Washington State University. From Corbin et al., 2013.
How are biodegradable plastic mulches produced?Many biodegradable plastic mulches that are commercially available are films made from plant starch; these are prepared using conventional plastics processing technology. However, due to the poor mechanical properties of starch, including its brittleness, starch must be blended with other polymers and/or plasticizers. Products currently on the market that contain plant starch include Biomax TPS (DuPont, USA), Biopar (Biop, Germany), Paragon (Avebe, Netherlands), BiosafeTM (Xinfu Pharmaceutical Co., China), Eastar BioTM (Novamont, Italy), Eco-Flex® (BASF, Germany), Ingeo® (NatureWorks, USA) and Mater-Bi® (Novamont, Italy) (Hayes et al., 2012).
Two polymers that may have a future role in biodegradable plastic mulches are polylactic acid (PLA) and polyhydroxyalkanoate (PHA). PLA is a highly versatile, biodegradable polyester derived from 100% renewable resources such as corn and sugar beet starch, and offers great promise in a wide range of commodity applications (Drumright et al., 2000). Starch is converted by microorganisms into lactic acid through fermentation. Lactic acid molecules are then linked together into long chains called polymers. PLA is a relatively inexpensive biopolymer to manufacture (~ $0.95 per lb), and can be produced in large quantities (Endres and Siebert-Raths, 2011). The PLA polymer is highly attractive for biological and medical applications because it can be spun into filaments that can be used to make textiles or films (Gupta et al., 2007). PHAs are promising biodegradable plastics that have been highlighted as "green" polymers because they are made from renewable resources in a one-step process by the bacterial fermentation of sugars and/or lipids (Kaihara et al., 2005; Posada et al., 2011; Hayes et al., 2012). PHA polymers may be produced from microbes or plants; but currently, microbes are the primary source (Keshavarz and Roy, 2010).
New experimental agricultural mulches have been prepared from PLA and PHA blends using nonwovens textile technology (Wadsworth et al., in press). Nonwovens are manufactured sheets, webs or bats (wadded rolls) of directionally or randomly oriented fibers or filaments, bonded together. Nonwovens may be manufactured by spunbond or meltblown processes. In the spunbond process, polymers are first melted and then extruded through spinnerets, producing filaments which are cooled and laid down on a conveyer belt to form a web. In the meltblown process, polymers are extruded through a die or spinneret, and the filaments are stretched, dispersed, cooled, and then collected on a roll. Generally, meltblown nonwovens have smaller fiber sizes and have lower mechanical strength than spunbond nonwovens (Hayes et al., 2012).
Some processes that are used to form biodegradable polymers utilize additives, such as nucleating agents (chemical substances incorporated in plastics for the growth of crystals in the polymer melt), plasticizers, coloring agents, performance additives, and/or lubricants to improve the mechanical properties of the plastic. The environmental impact of many additives may be a major concern in organic as well as conventional crop production. Some additives are derived from petroleum and/or are chemically processed, and are therefore considered synthetic material by National Organic Program (NOP) standards (Hayes et al., 2012; NOS, 2012), which has prevented their use in U.S. organic agriculture. In addition, the NOP considers PLA to be synthetic because PLA is chemically polymerized (Briassoulis and Dejean, 2010). While the PHA polymer is made directly by microorganisms in the fermentation process (thereby considered "natural" by the NOP), it is highly crystalline, making its end product more brittle, and less desirable, unless blended with PLA or other co-polymers (Hayes et al., 2012).
What constitutes biodegradability?Many agricultural plastics are advertised as "biodegradable"; however, such claims need to be evaluated carefully. For a manufacturer to employ the claim of biodegradability, a set of specified standards need to be met. ASTM International (formerly known as the American Society for Testing and Materials) has prepared a series of standards for "compostable plastics" to measure biodegradability under municipal or industrial composting conditions, referred to as ASTM D6400.
The ASTM D6400 specification encompasses several ASTM standardized tests, such as the "inherent biodegradability" of the plastic material via ASTM D5988-03. This test measures the microbial conversion of the plastic’s carbon (C) atoms to carbon dioxide (CO2), over time. A standard that is embedded in ASTM D6400 specifies that 90% of C atoms must be mineralized, that is, converted to CO2 within 180 days by microorganisms (ASTM, 2003). In the laboratory, CO2 release is measured through a relatively inexpensive titration method.
Biodegradability-related standards are comprised of criteria that address the following three issues:
(i) The conditions of the system —industrial-scale composting or anaerobic digestion, soil, marine, etc.
(ii) The time frame—number of days for carbon molecules in plastic to be converted to CO2.
(iii) The fraction of carbon atoms that are to be fully mineralized by microorganisms – generally expected to be at least 90%.
Many mulches claiming to be "biodegradable" are actually "compostable", i.e., able to fulfill the requirements of ASTM D6400, or related standards. Moreover, no standard currently exists for measuring the biodegradability of plastics incorporated into soil under field conditions. To meet this need for measuring biodegradability within the soil, ASTM International is developing a new standard (Work Item 29802) entitled Aerobically Biodegradable Plastics in the Soil Environment (ASTM, 2012). In this new standard, biodegradable mulches must break down into CO2, water and environmentally benign substances within one or two years, leaving no harmful residues. The ability of existing and emerging biodegradable plastic mulch films to meet these criteria in the soil environment has been the topic of several investigations (Harding et al., 2007; Hayes et al., 2012; Miles et al., 2012; Hoshino et al., 2007; Kapanen et al., 2008; Kijchavengkul et al., 2008; Kyrikou and Briassoulis, 2007; Mohee and Unmar, 2007; Tachibana et al., 2009; Wadsworth et al., 2009), and continues to be researched.
Evaluating degradation/deterioration vs. biodegradationThe term degradation is used to denote changes in physical properties caused by chemical reactions involving bond scission in the macromolecule (polymer). Biopolymer degradation includes changes of physical properties, caused not only by chemical reactions, but also by physical forces. Because the term polymer degradation involves a deterioration in the functionality of polymeric materials, "degradation" and "deterioration" are often used interchangeably (Schnabel, 1992). ASTM and the International Organization for Standardization (ISO) define degradation as an irreversible process leading to a significant change of the structure of a material, typically characterized by a loss of properties (e.g. integrity, molecular weight, structure or mechanical strength) and/or fragmentation, as affected by environmental conditions, proceeding over a period of time, and comprising one or more steps (Krzan et al., 2006).
The efficiency of the plastic degradation process varies by environment and may also be affected by the concentration of chemicals present that may react with the plastic. Environmental factors such as temperature, moisture level, atmospheric pressure, concentrations of acids and metals, and light exposure all have an effect on the rate of degradation that is due to microorganisms i.e., biodegradation (Kyrikou and Briassoulis, 2007). However, weight loss and other physical, chemical and mechanical property reductions in biodegradable plastic do not comprise the full measure of percent biodegradation unless microbial utilization of C (via CO2 conversion) is also measured. Percent biodegradation is the measure of the rate and amount of CO2 released from the total C input (from the mulch), and is a direct measure of the amount of C being utilized by the microbial community (Narayan, 2010).
While biodegradation measurements in the field or laboratory are relatively straightforward for well equipped and trained scientists, they are impractical for farmers to perform. Until the scientific community and the NOP can provide farmers with repeatable results on field performance of biodegradable plastic mulch products that are recommended for organic use, it may be advisable for farmers to monitor the degree of mulch degradability (see limitations below). Miles and others (2012) assessed percent visual deterioration (PVD) of biodegradable plastic mulch under field conditions and counted the number of rips, tears and holes (RTH) in a designated portion of the mulch twice per month. PVD proved to be a fair assessment of mulch deterioration while RTH did not. Cowan (personal communication) evaluated PVD during two late-summer broccoli seasons, and then measured mulch fragment recovery over the course of one year after mulches were tilled into the soil. At each of five sampling times, three random four-inch-diameter x six-inch-deep soil samples were collected (using a golf cup cutter) per 28-foot of mulch treated bed. Samples were sieved to retrieve mulch fragments,and photographs of mulch fragments were digitally analyzed to measure average area of individual fragments, fragment counts, and total fragment area. Findings indicated that post-tillage mulch recovery can be measured using this method. Two of the mulch products evaluated, Crown 1 and BioAgri, were recovered at 0% and 34%, respectively, within 13 months after soil incorporation.
Moore-Kucera et al., (in prep.) as part of a three-year field study, buried mesh bags containing a 4 inch square of biodegradable plastic mulch (previously weathered in the field for one growing season) and 300-400 grams of native agricultural soil four inches deep in field plots at three locations (Knoxville, TN, Lubbock, TX, and Mount Vernon, WA). At each location, one mesh bag was removed from replicated plots every 6 months for 24 months and the residual mulch pieces were evaluated for percent surface area remaining (Figure 3). Results varied widely by location, each with unique physical (light, temperature, moisture, wind, etc.), chemical (pH) and soil microbial attributes (Corbin et al., 2013; Bailes et al., in press; Moore-Kucera, 2012). For further information on experimental mulch degradation in the field, see the Washington State University Factsheet Using Biodegradable Plastics as Agricultural Mulches (Corbin et al., 2013). Although these types of field measurements will not determine percent mulch biodegradation per se, they are relatively simple sampling procedures that farmers, Extension or Agricultural Agency personnel can perform without the use of laboratory equipment, and provide a visual estimate of degradation/deterioration and mulch recovery in the field.
Figure 3. Samples of starch-based biodegradable plastic mulch (BioTELO®) recovered after twenty-four months burial in the field at three experimental locations. Photo credit: J. Moore-Kucera, Texas Tech University.
Biodegradable plastic mulch limitations in certified organic productionIn the U.S., organic crop producers have not been able to use currently available biodegradable plastic mulch products because these products did not conform to NOP standards. To be acceptable for organic production, biodegradable plastic mulch must be entirely composed of constituents derived from natural resources (bio-based), cannot contain synthetics such as petroleum-derived ingredients or additives, and cannot be chemically modified during the manufacturing process (NOS, 2012; Corbin et al., 2013; Corbin et al., 2009).
An additional requirement to meet the organic standards is that feedstocks, such as corn, used to produce the polymer, must be free of genetically modified organisms (GMOs). Similarly, any polymer made from microbial fermentation, such as PHA, must be produced by organisms that have not been genetically modified. Biodegradable plastic mulch manufacturers must certify that their feedstocks and microbial fermentation processes are GMO-free; however, there is no available test to verify GMO byproducts in the final product. While there is no specific NOP policy on GMOs in biodegradable plastic feedstock, the NOP has developed an ad-hoc committee to clarify the GMO issue.
Another requirement for use in certified organic production is that the resins and any inert additives used in the processing and formulation of biodegradable plastic mulch products must be identified and compared to the National List of allowable substances. The designation of ingredients as "proprietary" is not adequate for review and approval by the National Organic Standards Board (NOSB) – the sole authority to recommend adding or removing materials from the National List of acceptable and prohibited substances.
Finally, any biodegradable plastic mulch that may eventually be approved for use by the NOP must completely biodegrade into carbon dioxide, water, and microbial biomass within a "reasonable" timeframe without forming harmful residues or by-products. Sufficient data will be needed to verify that each biodegradable mulch is truly biodegradable in an agricultural system.
A growing contingency of manufacturers and organic farmers who wanted this interpretation of the NOP standards to be revised so that biodegradable plastic mulches are included on the National List of allowable "synthetic" substances has recently gained recognition (NOSB, 2012). As a precedent, the organic standards in Canada and the European Union (E.U.) have allowed some use of currently available biodegradable plastic mulches. In 2012, the Biodegradable Products Institute (BPI) petitioned the NOP to allow the addition of biodegradable plastic mulch under section 205.206 (c) of the National Organic Standards Biodegradable Plastic Mulch Made from Bioplastics: without removal at the end of the growing or harvest season (BPI, 2012). In October 2012, the NOSB voted 12-3 to formally recommend the NOP approve biodegradable plastic mulch in organic production (NOSB, 2012). Included in this recommendation is a requirement that organic growers take appropriate actions to ensure complete degradation (NOSB, 2012) of biodegradable plastic mulch products on their farms, underlining the importance of evaluating on-site degradation. As of 2013, the recommendation has yet to be accepted and defined by the NOP as a rule, so while these materials may be allowable in the near future, current use of biodegradable plastic mulch in U.S. certified organic production remains prohibited.
Glossary of Key TermsAbiotic Oxidation - nonliving substances or environmental factors combined with oxygen to cause a loss of electrons.
Aldehydes - Compounds RC(=O)H , in which a carbonyl group (a functional group composed of a carbon atom double-bonded to an oxygen atom) is bonded to one hydrogen atom and to one R group or side chain (IUPAC, 1997).
Biodegradable plastic - Degradable plastic in which the degradation results from the action of naturally occurring microorganisms such as bacteria, fungi, and algae (ASTM, 2011; 2004).
Bio-based - Commercial or industrial products (other than food or feed) that are composed in whole or in significant part of biological products or renewable domestic agricultural materials (including plant, animal, and marine materials) or forestry materials (Biobased US, 2007).
Bioplastics - Form of plastics derived from renewable biomass sources, such as vegetable fats, oils or starches.
Bond scission - breakage of a chemical bond, especially one in a long chain molecule (Oxford Dictionary, 2013).
Deterioration - To weaken or disintegrate.
Degradation - the breakdown of an organic compound.
Feedstock - Raw material that is used to supply or fuel a process.
Fermentation - The process in which cells (microorganisms, plant or animal cells) are cultured in a bioreactor in liquid or solid medium to convert organic substances into biomass (growth) or into products (IUPAC, 1997).
Genetically Modified Organism (GMO) - An organism whose genetic material has been altered using genetic engineering techniques; also referred to as a genetically engineered organism (GEO).
Inert - Stable and unreactive under specified conditions (IUPAC, 1997).
Ketones - Compounds in which a carbonyl group (a functional group composed of a carbon atom double-bonded to an oxygen atom) is bonded to two carbon atoms. (IUPAC, 1997).
Microbial biomass - Material produced by the growth of microorganisms (IUPAC, 1997).
Mineralization - Microbial conversion of organic matter into inorganic substances, such as water and carbon dioxide (Guggenberger, 2005).
PHA - Polyhydroxyalkanoates (PHAs) are a group of biologically synthesized polyesters that are considered promising eco-efficient bioplastics because they are both biobased and biodegradable, thus meeting the criteria of a closed loop life cycle (Reis et al., 2011).
PLA - polylactic acid (PLA) is a thermoplastic polymer made from lactic acid and has mainly been used for biodegradable products, such as plastic bags and planting cups, but in principle PLA can also be used as a matrix material in composites (Oksman et al., 2003).
Polyethylene - a polymer of ethylene; especially: any of various partially crystalline lightweight thermoplastics (CH2CH2)x that are resistant to chemicals and moisture.
Polymer - A molecule of high relative molecular mass, the structure of which essentially comprises the multiple repetition of units derived, actually or conceptually, from molecules of low relative molecular mass (IUPAC, 1997).
Polymerization - Any process in which relatively small molecules, called monomers, combine chemically to produce a very large chainlike or network molecule, called a polymer (Encyclopedia Britannica 2012).
Synthetic material (according to the USDA NOSB) - A substance that is formulated or manufactured by a chemical process or by a process that chemically changes a substance extracted from naturally occurring plant, animal, or mineral sources, except that such term shall not apply to substances created by naturally occurring biological processes (Sullivan, 2011).
References and Citations- ASTM International. 2012. Standard specification for aerobically biodegradable plastics in soil environment (ASTM WK29802), West Conshohocken, PA USA.
- ASTM D 5988-03. 2003. Standard test method for determining aerobic biodegradation in soil of plastic materials or residual plastic materials after composting. ASTM International, West Conshohocken, PA USA.
- ASTM D6400. 2004. International standard specification for compostable plastics. ASTM International, West Conshohocken, PA USA.
- ASTM D883. 2011. International standard terminology relating to plastics. ASTM International, West Conshohocken, PA USA.
- Bailes, G., Lind, M., Ely, A., Powell, M., Moore-Kucera, J., Miles, C., Inglis, D., and Brodhagen, M. 201x. Isolation of native soil microorganisms with potential for breaking down biodegradable plastic films used in agriculture. Journal of Visualized Experiments (accepted Oct 10, 2012).
- BPI 2012. Support the BPI's Biodegradable Mulch Film Petition. [Online]. Biodegradable Products Institute, Inc. New York, NY. Available at: http://www.bpiworld.org (verified 30 April, 2013).
- Briassoulis, D.; Dejean, C. 2010. Critical Review of Norms and Standards for Biodegradable Agricultural Plastics Part I: Biodegradation in Soil. Journal of Polymers and the Environment. 18 (3), 384-400. Available online at: http://openagricola.nal.usda.gov/Record/IND44454056 (verified 30 April, 2013).
- Environmental Protection Agency. 2011. Outdoor Air: Industry, Business, and Home Backyard Trash Burning. [Online]. Available at: http://www.epa.gov/oaqps001/community/details/barrelburn.html (verified 30 April, 2013).
- Corbin, A., Miles, C., Hayes, D., Dorgan, J., and Roozen, J. July 25–28, 2009. Suitability of Biodegradable Plastic Mulches In Certified Organic Production. American Society of Horticulture Conference, St. Louis, Missouri. Available online at: http://ashs.org/db/horttalks/detail.lasso?id=684 (verified 30 April, 2013).
- Corbin, A.T., Miles, C., Cowan, J., Hayes, D., Dorgan, J., and D. Inglis. 2013. Using Biodegradable Plastics as Agricultural Mulches. Washington State University Extension Fact Sheet FS103E; 6pp. Available online at http://cru.cahe.wsu.edu/CEPublications/FS103E/FS103E.pdf (verified 30 April, 2013).
- Drumright, R. E., P. R. Gruber & D. E. Henton 2000. Polylactic acid technology. Advanced Materials 12, 1841-1846. Available online at: (verified 30 April, 2013).
- Encyclopedia Britannica 2012. Encyclopedia Britannica Online Academic Edition [Online]. Encyclopedia Britannica Inc. Web. 18 Sep. 2012. Available at: http://www.britannica.com/ (verified 30 April, 2013).
- Endres, H.-J.; Siebert-Raths, A., 2011. Engineering Biopolymers - Markets, Manufacturing, Properties and Applications. Hanser Publishers: Munich.
- Garthe, J.W. and P.D. Kowal. 1993. Recycling used agricultural plastics. Penn State Fact Sheet C-8. Available online at http://pubs.cas.psu.edu/freepubs/pdfs/c8.pdf (verified 30 April, 2013).
- Guggenberger, G. 2005. Microorganisms and soil genesis. In Microorganisms in soils: roles in genesis and functions, Buscot, F.; Varma, A., Eds. Springer-Verlag: Berlin; pp 85-106.
- Gupta, B., N. Revagade & J. Hilborn 2007. Poly(lactic acid) fiber: An overview. Progress in Polymer Science 32, 455-482. Available online at http://dx.doi.org/10.1016/j.progpolymsci.2007.01.005 (verified 30 April, 2013).
- Hakkarainen, M.; Albertsson, A.-C. 2004. Environmental degradation of polyethylene. Advances in Polymer Science 169 (Long-Term Properties of Polyolefins), 177-199. Available online at: http://link.springer.com/chapter/10.1007%2Fb13523 (verified 30 April, 2013).
- Harding, K. G., J. S. Dennis, H. von Blotnitz & S. T. L. Harrison 2007. Environmental Analysis of Plastic Production Processes: Comparing Petroleum-Based Polypropylene and Polyethylene with Biologically-Based Poly-B-Hydroxybutyric Acid Using Life Cycle Analysis. Journal of Biotechnology 130, 57-66. Available online at: http://www.ncbi.nlm.nih.gov/pubmed/17400318 (verified 30 April, 2013).
- Hayes, D. G., S. Dharmalingam, L. C. Wadsworth, K. K. Leonas, C. A. Miles & D. A. Inglis. 2012. Biodegradable Agricultural Mulches Derived from Biopolymers. In Degradable Polymers and Materials, Principles and Practice, ed. A. I. Kishan C. Khemani, Carmen Scholz, University of Alabama at Huntsville. ACS Books.
- Hoshino, A., M. Tsuji, M. Momochi, A. Mizutani, H. Sawada, S. Kohnami, H. Nakagomi, M. Ito, H. Saida, M. Ohnishi, M. Hirata, M. Kunioka, M. Funabash & S. Uematsu 2007. Study of the determination of the ultimate aerobic biodegradability of plastic materials under controlled composting conditions. Journal of Polymers and the Environment 15, 275-280. Available online at: http://link.springer.com/article/10.1007%2Fs10924-007-0078-z (verified 30 April, 2013).
- IUPAC. 1997. Compendium of Chemical Terminology, 2nd ed. (the "Gold Book"). Compiled by A. D. McNaught and A. Wilkinson. Blackwell Scientific Publications, Oxford. XML Created by M. Nic, J. Jirat, B. Kosata; updates compiled by A. Jenkins. ISBN 0-9678550-9-8. Available online at: http://goldbook.iupac.org/ (verified 30 April, 2013).
- Kaihara, S., Y. Osanai, K. Nishikawa, K. Toshima, Y. Doi & S. Matsumura 2005. Enzymatic transformation of bacterial polyhydroxyalkanoates into repolymerizable oligomers directed towards chemical recycling. Macromolecular Bioscience 5, 644-652. Available online at: http://www.ncbi.nlm.nih.gov/pubmed/15988790 (verified 30 April, 2013).
- Kapanen, A., E. Schettini, G. Vox & M. Itavaara 2008. Performance and Environmental Impact of Biodegradable Films in Agriculture: A Field Study on Protected Cultivation. Journal of Polymers and the Environment 16, 109-122. Available online at: http://link.springer.com/article/10.1007%2Fs10924-008-0091-x (verified 30 April, 2013).
- Keshavarz, T. & I. Roy 2010. Polyhydroxyalkanoates: bioplastics with a green agenda. Current Opinion in Microbiology 13, 321-326. Available online at: http://dx.doi.org/10.1016/j.mib.2010.02.006 (verified 30 April, 2013).
- Kijchavengkul, T., R. Auras, M. Rubino, M. Ngouajio & R. T. Fernandez 2008. Assessment of aliphatic-aromatic copolyester biodegradable mulch films. Part I: Field study. Chemosphere 71, 942-953. Available online at: http://www.ncbi.nlm.nih.gov/pubmed/18262221 (verified 30 April, 2013).
- Krzan, A., S. Hemjinda, S. Miertus, A. Corti and E. Chiellini. 2006. Standardization and certification in the area of environmentally degradable plastics. Polymer Degradation and Stability 91:2819-2833. Available online at: http://dx.doi.org/10.1016/j.polymdegradstab.2006.04.034 (verified 30 April, 2013).
- Kyrikou, I. & D. Briassoulis 2007. Biodegradation of agricultural plastic films: A critical review. Journal of Polymers and the Environment 15, 125-150. Available online at: http://link.springer.com/article/10.1007%2Fs10924-007-0053-8 (verified 30 April, 2013).
- Lemieux, P. M. 1997. Evaluation of emissions from the open burning of household waste in barrels. US Environmental Protection Agency Report 600/R-97-134a; Washington, DC; p 70. Available online at: http://www.ecy.wa.gov/programs/air/outdoor_woodsmoke/PDFs/EPAbarlbrn1.pdf (verified 30 April, 2013).
- Levitan, L. 2005. Reducing dioxin emissions by recycling agricultural plastics: Creating a viable alternative to open burning. In Great Lakes Regional Pollution Prevention Roundtable, New York.
- Miles, C., R. Wallace, A. Wszelaki, J. Martin, J. Cowan, T. Walters, and D. Inglis. 2012. Durability of potentially biodegradable alternatives to plastic mulch in three tomato production regions. HortScience 47(9):1270-1277. Available online at: http://hortsci.ashspublications.org/content/47/9/1270.abstract (verified 30 April, 2013).
- Mohee, R. & G. Unmar 2007. Determining biodegradability of plastic materials under controlled and natural composting environments. Waste Management 27, 1486-1493. Available online at: http://dx.doi.org/10.1016/j.wasman.2006.07.023 (verified 30 April, 2013).
- Moore-Kucera, J., M. Davinic, L. Fultz, J. Lee, C.A. Miles, M. Brodhagen, J. Cowan, R.W. Wallace, A. Wszelaki, J. Martin, J. Roozen, B. Gundersen and D.A. Inglis. 2011. Biodegradable Mulches: Short-term degradability and impacts on soil health. HortScience 46(10):S68.
- Narayan, R. 2010. Misleading claims and misuse of standards proliferate in the nascent bioplastics industry space. Bioplastics Magazine, Polymedia Publisher GmbH. 10(1):38-41. Available online at: http://www.bioplasticsmagazine.com/bioplasticsmagazine-wAssets/docs/arti... (verified 30 April, 2013).
- National Organic Standards (NOS) 2012. § 205.206 Crop pest, weed, and disease management practice standard. § 205.601(b)(2)(i-ii) Synthetic substances allowed for use in organic crop production. Available online at: http://www.ecfr.gov/cgi-bin/text-idx?c=ecfr&sid=3f34f4c22f9aa8e6d9864cc2... (verified 30 April, 2013).
- National Organic Standards Board (NOSB) 2012. Formal recommendation to classify Biodegradable biobased mulch film as synthetic and Petition to list Biodegradable biobased mulch films on §205.601(b)(2) Mulches. http://www.ams.usda.gov/AMSv1.0/getfile?dDocName=STELPRDC5101277 (verified 30 April, 2013).
- Oksman K, Skrifvars M, Selin JF (2003) Natural fibres as reinforcement in polylactic acid (PLA) composites. Composites Science and Technology 63: 1317-1324. Available online at: http://dx.doi.org/10.1016/S0266-3538(03)00103-9 (verified 30 April, 2013).
- Oregon Administrative Rules (OAR) 2013. Department of Environmental Quality, Rules for Open Burning, chapter 340, division 264, rule 0010 (short form: OAR 340-264-0010). Available online at: http://www.deq.state.or.us/aq/burning/openburning/openburn.asp (verified 30 April, 2013).
- Oxford Dictionaries 2013. [Online]. Copyright © 2013 Oxford University Press. Available at: http://oxforddictionaries.com/definition/english/scission (verified 30 April, 2013).
- Posada, J. A.; Naranjo, J. M.; Lopez, J. A.; Higuita, J. C.; Cardona, C. A. 2011. Design and analysis of poly-3-hydroxybutyrate production processes from crude glycerol. Process Biochem. (Amsterdam, Neth.) 46 (1), 310-317. Availale online at: http://dx.doi.org/10.1016/j.procbio.2010.09.003 (verified 30 April, 2013).
- Reis M, Albuquerque M, Villano M, Majone M (2011) 6.51 - Mixed Culture Processes for Polyhydroxyalkanoate Production from Agro-Industrial Surplus/Wastes as Feedstocks. In Comprehensive Biotechnology (Second Edition), Editor-in-Chief: Murray M-Y (ed), pp 669-683. Burlington: Academic Press.
- Schnabel, W. 1992. Polymer Degradation. Hanser Publishers, Munich, Germany, pg. 1.
- Schonbeck, M.W. and G.K. Evanylo. 1998. Effects of mulches on soil properties and tomato production: I. Soil temperature, soil moisture and marketable yield. Journal of Sustainable Agriculture 13:55–81. Available online at: http://www.tandfonline.com/doi/abs/10.1300/J064v13n01_06#preview (verified 30 April, 2013).
- Shogren, R.L. and Hochmuth, R.C., 2004. Field evaluation of watermelon grown on paper-polymerized vegetable oil mulches. HortScience 39:1588-1591. Available online at: http://hortsci.ashspublications.org/content/39/7/1588.full.pdf (verified 30 April,, 2013).
- Smith, B. R., L. C. Wadsworth, M. G. Kamath, A. Wszelaki & C. E. Sams. 2008. Development of Next Generation Biodegradable Mulch Nonwovens to Replace Polyethylene Plastic. In International Conference on Sustainable Textiles (ICST 08). Wuxi, China (CD ROM).
- Sullivan, D. 2011. Compostable plastics and organic farming. BioCycle (3), 36-41. Available online at: http://www.biocycle.net/2011/03/compostable-plastics-and-organic-farming/ (verified 30 April, 2013).
- Tachibana, Y., T. Maeda, O. Ito, Y. Maeda & M. Kunioka. 2009. Utilization of a Biodegradable Mulch Sheet Produced from Poly(Lactic Acid)/Ecoflex®/Modified Starch in Mandarin Orange Groves. International Journal of Molecular Sciences 10, 3599-3615. Available online at http://www.ncbi.nlm.nih.gov/pmc/articles/PMC2758133/ (verified 30 April, 2013).
- United States Department of Agriculture Federal Biobased Products Preferred Procurement Program (FB4P). 2007. What are bio-based products? [Online]. Available at: http://www.dm.usda.gov/procurement/programs/biobased/awarenessbrochure_m... (verified 30 April, 2012).
- Wadsworth, L. C., A. Wszelaki, D. G. Hayes & B. R. Smith. 2009. Development of Enhanced Biodegradable Mulch Nonwovens to Replace Plastic Films. AATCC International Conference, Myrtle Beach, SC, March 10-12, 2009.
- Wadsworth, L. C., Hayes, D. G., Wszelaki, A. L., Washington, T. L., Martin, J., Lee, J., Raley, R., Pannell, C. T., Dharmalingam, S., Miles, C., Saxton, A., and Inglis, D. A. 201x. Evaluation of degradable spun-melt 100% polylactic acid nonwovens mulch materials in a greenhouse environment. Journal of Engineered Fibers and Fabrics (accepted Sep 20, 2012).
This is an eOrganic article and was reviewed for compliance with National Organic Program regulations by members of the eOrganic community. Always check with your organic certification agency before adopting new practices or using new materials. For more information, refer to eOrganic's articles on organic certification.
eOrganic 8260
Direct Marketing Channels & Strategy for Organic Products
eOrganic author:
Garry Stephenson, Oregon State University
Farmers approach direct marketing in a variety of ways using single or multiple channels. The goal generally is to develop a strategy to sell all the product they produce. This can be through one marketing channel or several. Farms may also add additional direct market channels as the business grows. For instance, many farmers begin with selling through a farmers’ market or a roadside stand. As the business grows they can add other direct channels such as a CSA, grocery or restaurant sales. Direct sales channels for specific crops or a segment of a crop may be combined with wholesale channels. The options are nearly endless.
Farmers' market in Portland, Oregon. Photo credit: Garry Stephenson, Oregon State University
There are many approaches to farm direct marketing. Some have been around for decades others are have been developed more recently. The most common types of direct marketing are:
- Community Supported Agriculture (CSA)
- Farmers’ Markets
- U-Pick farms
- Farm Stands
- Restaurants
- Farm to School and Institutions
- Agritourism
- Online Marketing
Community supported agriculture is a relatively recent and innovative concept that is intended to create a relationship between farmers and consumers wherein risks and bounties are shared. CSA customers buy shares for a season by paying a fee in advance. In return, they receive a regular (in most cases weekly) selection of food. Having cash in advance of the growing season and a regular customer following provide financial security for farmers. The regular supply of food grown on the farm provide nutritional security and a sense of community for customers. On some farms, get-togethers with customers or workdays are part of the agreement. In its purest form, customers share in the risk of low production and crop failures, as well as any abundance, by receiving less or more food. This aspect has seen a variety of adaptations on CSA farms.
Generally, families pay about $400 to $600 per share. Operating a CSA requires excellent crop management skills to provide attractive and diverse weekly food baskets, as well as good customer service. CSA can be integrated with farmers’ market sales and other techniques. It has been an excellent start-up strategy for many small organic farms, providing crucial cash flow at the beginning of the growing season and allowing farmers to “boot strap” their way into farming.
CSA Resources- Robyn's resources for CSA's [Online]. Robyn Van En Center. Wilson College. Available at: http://www.wilson.edu/robyns-resources
- Food Network: Community Supported Agriculture [Video]. Cooking Up a Story. http://cookingupastory.com/show/food-network-community-supported-agricul... (verified 5 Mar 2010).
"Community Supported Agriculture (CSA) offers a holistic approach to the production of sustainable food grown in urban areas. In this story, we learn of one urban farmer who successfully uses the CSA model to produce long-term sustainable crops that are fresh, cost efficient to produce, environmentally friendly, and that offers local members a stake in the harvest crops." - Community supported agriculture in California, Oregon and Washington: Challenges and opportunities. R. Strochlic and C.Shelley. 2004. California Institute for Rural Studies (Available online at: http://www.cirsinc.org/Documents/Pub0504.1.pdf) (verified 5 Mar 2010).
- Community Supported Agriculture - LocalHarvest. LocalHarvest, Inc. Avabilable at: http://www.localharvest.org/csa/ (verified 5 Mar 2010).
A farmers’ market is a place where a number of growers assemble on a particular day to sell farm products directly to consumers. The sites are often parking lots, streets closed during the market, parks, etc. Farmers at these markets sell their products from “stands” that may consist of the back of a farm truck or a simple tabletop to elaborate and attractive covered displays.
Farmers generally receive retail prices or higher for their products. Start up costs for becoming involved in a farmers’ market can be very inexpensive—just a stall fee in some instances. Because of the low start up investment, farmers’ markets can provide a low risk setting for new farmers or an opportunity to try out new products. Many farmers participate in more than one market to increase their sales.
Farmers’ markets also provide the opportunity to build a customer base. Some farms advertise other outlets for buying their products (other farm direct marketing methods, or retail stores for instance).
Farmers’ Market Resources- Tools for rapid market assessments. L. Lev, L. Brewer, and G. Stephenson. 2008. Oregon State University Extension Service. (Available at: http://smallfarms.oregonstate.edu/sites/default/files/small-farms-tech-report/eesc_1088-e.pdf) (verified 5 Mar 2010).
Most farmers’ markets lack information to make effective changes and improvements. This publication by Larry Lev, Linda Brewer, and Garry Stephenson of Oregon State University describes three simple, low-cost methods to address the information gaps: attendance counts, dot surveys, and comments and observations. - Starting and operating a farmers' market: Frequently asked questions [Online]. B. McKelvey, M.Hendrickson, and C. Weber. 2008. University of Missouri Extension.
Available at: http://extension.missouri.edu/explore/agguides/hort/g06223.htm (verified 5 Mar 2010).
From University of Missouri Extension, this guide is intended to be a resource for people who are either starting a new farmers' market or improving an existing market. The guide follows a frequently asked questions format, provides brief answers to each question and then directs readers to free, online publications that answer the questions in more detail. In addition to addressing questions faced by market organizers across the country, the guide includes information about legal and regulatory issues for farmers' market organizers in Missouri. - The art and science of farmers’ market display [Slide show]. Entrepreneurs and Their Communities Community of Practice. eXtension. Available at:http://www.extension.org/pages/10986/the-art-and-science-of-farmers-market-display#.U87YRBD5eTQ (verified 5 Mar 2010).
- Enhancing the success of northwest farmers’ markets. G. Stephenson, L. Lev, and L. Brewer. 2006. Oregon Small Farm Technical Report Number 22. Oregon State University Extension Service. Available at: http://smallfarms.oregonstate.edu/sites/default/files/TechReport22.pdf (verified 5 Mar 2010).
This executive summary of a large project examines the conditions associated with success and failure of individual farmers’ markets and provides information and recommendations for market organizers to assist with their decision making and strategic planning. It explores historic trends related to growth and decline in market numbers; it examines the management organization associated with markets of specific sizes; it looks at the characteristics and issues associated with markets that fail; and it synthesizes a model that illustrates how farmers’ market organizers successfully adapt to barriers and challenges in their environment.
U-Pick or Pick-Your-Own farms grow crops specifically to be harvested by customers. In this manner, the task of picking the crop, one of the higher costs of growing fruits and vegetables, is passed on to customers. U-Pick farms have traditionally appealed to families who do home canning. There continues to be an interest by families in picking produce for fresh use and, in some instances, having their children experience where their food comes from. As with many direct marketing techniques, U-Pick operations can be blended with other marketing techniques such as roadside markets, farmers’ markets, and so on.
Farm StandsFarm stands or markets are structures of some type from which the farm’s products are sold. They can range in sophistication from a stand with a coffee can for purchases by honor system to a building with refrigerated storage and several employees. They tend to be located on the farm, often on a well-traveled road with good access and parking. They can operate seasonally or all year and focus on one product or a full line of products. Roadside markets usually charge near retail prices. Given that farm stands or markets are structures, they are subject to local building codes and highway setback regulations.
RestaurantsMany farms are now marketing directly to restaurants providing the specific products and the high quality that chefs are demanding. Many restaurants cultivate relationships with farms even noting the farm name and its product on their menu. These restaurants serve a niche of customers who find high quality food produced locally appealing. Supporting local farms is a philosophical goal for these restaurants.
Similar opportunities for farm direct sales are to institutions that serve food to large or “captive” groups such as:
- Hospitals
- Retirement and nursing facilities
- More
- Selling to restaurants. J. Bachmann. 2004. National Center for Appropriate Technology (ATTRA). Available at: https://attra.ncat.org/attra-pub/download.php?id=266 (verified 24 May 2019).
"Upscale restaurants serving locally-grown produce are in the headlines nationwide. Growing for this market is both lucrative and demanding. Profiles of growers from around the country illustrate successful strategies and points to remember when working with chefs. A Spanish language version of this publication is available as Nuevos Mercados para Su Cosecha."
The Local Food Connection and Farmer-Chef Connection are programs that bring farmers and chefs together for a day of networking and deal making. Read Notes to a Farmer and a Chef to learn how farmers and chefs can best work with each other. The Farmer-Chef Connection is a project of the Portland Chefs Collaborative and the Local Food Connection is a project of the Cascade Pacific RC&D.
Tip SheetsSelling directly to restaurants, retailers and institutions can be a great way to expand your business and develop a reliable customer base. Created by Community Involved in Sustaining Agriculture (CISA), these tipsheets are designed to help farmers respond to the unique challenges in reaching out to and maintaining relationships with buyers.
- Tools for selling to restaurants, retailers and institutions: Keys to a successful relationship. 2008. Community Involved in Sustaining Agriculture. Available at: http://www.buylocalfood.org/upload/resource/relationship-building%20fina... (verified 5 Mar 2010).
- Tools for selling to restaurants, retailers and institutions: Pricing and Invoicing. 2008. Community Involved in Sustaining Agriculture. Available at: http://www.buylocalfood.org/upload/resource/Pricing%20and%20invoicing%20... (verified 5 Mar 2010).
- Bring local food to local institutions: A resource guide for farm-to-school and farm-to-institution programs. B.C. Bellows, R. Dufour, and J. Bachmann. 2003. National Center for Appropriate Technology (ATTRA). Available at: https://attra.ncat.org/attra-pub/download.php?id=261 (verified 4 Jun 2019).
This provides farmers, school administrators, and institutional food-service planners with contact information and descriptions of existing programs that have made connections between local farmers and local school lunchrooms, college dining halls, or cafeterias in other institutions. To help communities initiate similar programs, this publication includes: resource lists of publications on how to initiate and manage local food programs, funding and technical assistance sources, and provisions of the 2002 Farm Bill that support farm-to-school and other community food programs. - Farm to school. Urban and Environmental Policy Institute, Occidental College. Available at: http://www.farmtoschool.org/ (verified 5 Mar 2010).
"Farm to School programs are popping up all over the U.S. These programs connect schools with local farms with the objectives of serving healthy meals in school cafeterias, improving student nutrition, providing health and nutrition education opportunities that will last a lifetime, and supporting local small farmers." - Sustainable food purchasing guide, first ed. Yale Sustainable Food Project. Yale University. Available at: http://www.yale.edu/sustainablefood/purchasing_guide_002.pdf.pdf (verified 5 Mar 2010).
"In 2007, the Yale Sustainable Food Project received a SARE grant to write an expanded set of definitions for dining halls to use across the northeast. Unlike other guides, this one focuses on agricultural practices, because these practices are inseparable from nutrition and sustainability. This guide establishes best and worst practices in the field. It provides a list of questions you need to ask to get the very best product for your institution. It also offers helpful hints, so that you can learn from work that has been done." - Rethinking school lunch guide | Center for Ecoliteracy. Center for Ecoliteracy. Available at: http://www.ecoliteracy.org/programs/rsl-guide.html (verified 5 Mar 2010).
"The Rethinking School Lunch guide provides a planning framework that contains tools and creative solutions to the challenges of improving school lunch programs, academic performance, ecological knowledge, and the well-being of our children. In its chapters, experts and practitioners highlight goals and challenges, showcase success stories, and offer resources for further exploration." - Farm-to-cafeteria connections: Marketing opportunities for small farms in Washington state. K. Sanger and L. Zenz. 2004. Small Farm and Direct Marketing Program, Washington State Department of Agriculture. Available at: http://agr.wa.gov/Marketing/SmallFarm/docs/102-FarmToCafeteriaConnection... (verified 5 Mar 2010).
An extensive resource guide for those interested in starting farm-to-cafeteria programs at all levels, with information for food services, farmers and others. Includes case studies of programs and a list of resources. - Farm-to-college. Community Food Security Coalition. Available at: http://farmtocollege.org/ (verified 5 Mar 2010).
"Farm-to-college programs connect colleges and universities with producers in their area to provide local farm products for meals and special events on campus. These programs may be small and unofficial, mainly involving special dinners or other events, or they may be large and well-established, with many local products incorporated into cafeteria meals every day." - Farm to hospital: Supporting local agriculture and improving health care. Center for Food & Justice, a division of the Urban & Environmental
Policy Institute at Occidental College. Available at: http://www.foodsecurity.org/F2H_Brochure.pdf (verified 5 Mar 2010).
"This brochure introduces interested farmers and hospital food service departments to the ins and outs of developing partnerships between hospitals and local farms. Included are examples of ways hospitals can improve the food they offer, issues for farmers to consider if they are interested in selling products to area hospitals, and specific case studies of successful programs."
Agritourism appeals to customers who have a desire to visit a farm and experience its activities. As Americans lose family ties with agriculture, many are interested in maintaining some sort of contact with farming; especially for their children. This is a theme with most types of direct marketing but is a key feature of agritourism. There are a variety of approaches.
On-farm bed and breakfasts allow overnight stays to relax in a bucolic atmosphere or, in some instances, work on the farm. The concept of “farm stays,” popular in Europe, is catching on in the U.S. Hay rides to gather Halloween pumpkins or Christmas trees and other family-oriented activities are popular on farms. Other activities such as cattle drives attract customers who are willing to pay to experience a celebrated part of our past and present. Agritourism and entertainment techniques can work in both urbanized areas and very rural areas. As with many direct marketing techniques, people skills are crucial.
Online MarketingThe internet provides a convenient method to advertise the farm business, sell products, and communicate with customers. Most households have access to the internet in their homes. This is a potentially large market for specialty farm products.
Farms may advertise on the internet by developing their own web sites or by participating in web-based farm directories. Farm homepages are an effective means for informing customers of products the farm grows, when they are available, and how to obtain them. Related blogs report on-farm or family activities. A farm may offer details on its CSA or identify which farmers’ markets it sells at. These types of web pages allow customers to see the farm and the people who work there. This enhances the personal aspect of farm direct marketing that many people find appealing. There are also opportunities for sales via the internet. Value-added or even fresh food products may be shipped to customers throughout the country. The internet is a quick and easy method for communicating with established customers. The latest information on product availability, farm news, and other information may be distributed to customers through an email list.
Online Marketing Tip SheetsLooking to create or improve your online marketing? Download one of these four tip sheets for pointers and guidance. They're full of useful tips and real-life experiences from local farmers. This series was created by Community Involved in Sustaining Agriculture (CISA).
- Creating a farm web site [Online]. Community Involved in Sustaining Agriculture. Available athttp://buylocalfood.org/upload/resource/CISA_TipSheets_WebSites.pdf ">: http://buylocalfood.org/upload/resource/CISA_TipSheets_WebSites.pdf (verified 5 April 2011).
- Online stores [Online]. Community Involved in Sustaining Agriculture. Available at http://buylocalfood.org/upload/resource/CISA_TipSheets_Stores.pdf (verified 5 April 2011).
- Blogs [Online]. Community Involved in Sustaining Agriculture. Available at http://buylocalfood.org/upload/resource/CISA_TipSheets_Blogs.pdf (verified 5 April 2011).
- Email Newsletters [Online]. Community Involved in Sustaining Agriculture. Available at http://buylocalfood.org/upload/resource/CISA_TipSheets_PermissionMarketing.pdf (verified 5 April 2011).
This is an eOrganic article and was reviewed for compliance with National Organic Program regulations by members of the eOrganic community. Always check with your organic certification agency before adopting new practices or using new materials. For more information, refer to eOrganic's articles on organic certification.
eOrganic 1493
Records Needed for Organic Poultry Certification
eOrganic authors:
Devon Patillo, CCOF
Dr. Jacquie Jacob Ph.D., University of Kentucky
Organic poultry producers are required to keep records to demonstrate compliance with USDA National Organic Program (NOP) requirements. Depending on the scale of operation and the method by which eggs, meat, or live birds are sold, certain records may be more appropriate than others.
Organic certification requirements allow recordkeeping systems to be adapted to a particular operation. However, all operations must keep records in enough detail to demonstrate to organic inspectors and certifiers that all requirements of the organic regulations are met. Recommended records are described below. Your certifier will determine your compliance with National Organic Program standards. Be prepared to work with your certifier and adjust your recordkeeping system to meet the required standards.
Poultry producers must meet the organic regulations summarized below. To read the full text of the organic regulations, visit The Electronic Code of Federal Regulations (e-CFR).
§ 205.103 Recordkeeping by certified operations
Below is the full text of the recordkeeping requirement of the National Organic Program.
(a) A certified operation must maintain records concerning the production, harvesting, and handling of agricultural products that are or that are intended to be sold, labeled, or represented as “100 percent organic,” “organic,” or “made with organic (specified ingredients or food group(s)).”(b) Such records must:
(1) Be adapted to the particular business that the certified operation is conducting;
(2) Fully disclose all activities and transactions of the certified operation in sufficient detail as to be readily understood and audited;
(3) Be maintained for not less than 5 years beyond their creation; and
(4) Be sufficient to demonstrate compliance with the Act and the regulations in this part.
(c) The certified operation must make such records available for inspection and copying during normal business hours by authorized representatives of the Secretary, the applicable State program's governing State official, and the certifying agent.
§ 205.236: Animal Origin
Section 205.236(c) requires that, “the producer of an organic livestock operation must maintain records sufficient to preserve the identity of all organically managed animals and edible and non-edible animal products produced on the operation.”
-
Animal purchase records
Birds must be managed organically starting no later than the second day of life. Keep records to show: date(s) of purchase, number of birds purchased, and the age of animals at time of purchase. -
Mortality and cull records
These records ensure that non-organic birds are not added to your flock. Document observed deaths and intentional culls. Bird deaths and culls subtracted from the number of purchased birds need to correspond to the number of birds being certified. Your certifier may use this information to list the number of birds on your certificate and to ensure that non-organic birds have not been added to your flock. (Purchases – Mortality & Culls = Size of current flock)
This means that all organic poultry must be grouped in flocks or otherwise identified, with corresponding records maintained of all feeds and feed supplements purchased and consumed for all stages of life; all health events and medications or activities; housing and pasture rotations; etc. Records must also be maintained of all products produced, including meat and eggs, or feathers for organic fishing flies.
§ 205.237: Feed-
Feed Production or Purchase Records
All feed provided must be certified organic. Feed production and/or purchase records need to demonstrate that all feed provided was certified organic. Keep copies of all purchase documentation. Ensure that the total number of pounds purchased is easy to determine. Feed and feed supplement records should include type of feed purchased, quantity purchased, dates purchased, source, and agency that certified the feed as organic. If mixing your own feed, you need to document the same information for each feed ingredient used. You need to list all supplements, including vitamins, amino acids, minerals, etc. used and the reason they were used. -
Feed labels, ingredient statements, and certification information
Keep documentation to demonstrate that the feed you purchased was in fact organic. Keep retail labels that include a “Certified Organic by [certifier’s name]” statement. These will appear under the manufacturer’s name on the retail label. If purchasing bulk feed without a retail label, you will need to obtain a list of ingredients in the feed formula and a copy of the manufacturer’s organic certificate, in addition to the purchase invoice and delivery ticket. -
Feed supplement and additive purchases
Only approved feed supplements and additives are allowed. Seek approval of feed supplements prior to use. Once approved, keep documentation of your purchases. Ensure the purchase records include the name of the product(s) purchased, quantity purchased, and date.
-
Health care product purchases (medicines, vaccines, drugs)
Only approved health care materials are allowed. Seek approval of any health care materials prior to use. Once approved, keep documentation of your purchases. Ensure the purchase records include the name of the product(s) purchased, quantity purchased, and date. -
Mortality/cull records
These records provide an indication of the health of your birds. Document observed deaths and intentional culls. If documented on a calendar, be sure to transfer the information from your calendar to a single location before your inspection so it will be easier to see trends or patterns.
A preventive health care program should include control of possible disease vectors including rodents, insects (e.g., external parasites, flies and darkling beetles), and internal parasites. The methods of control should be outlined with a record of monitoring and action(s) taken. Predator control measures should also be documented.
A preventive health care program also includes keeping equipment used clean as well as cleaning and disinfecting between flocks. A record must be kept of all sanitation and cleaning products used and when. Use of any vaccines must be recorded with the date used and source of the vaccine.
Any physical alterations used such as beak trimming, de-snooding, toe trimming, and wing trimming must be recorded with explanation of when performed and why.
As part of your biosecurity program you should limit visitors to your farm. If there are visitors, you should document who and when, and where they had been prior to the visit.
§ 205.239: Living conditions-
General information
Certifiers may require specific data about living conditions provided to birds. It is a good idea to have the following information available for your certifier. It is recommended to note the following information on a drawing of any coops or houses:- Perch space (inches per bird)
- Stocking density: Space per bird indoors (square feet per bird)
- Stocking density: Space per bird outdoors (square feet per bird)
- Number of birds per nest box (birds per nest box)
Organic poultry are required to have outdoor access depending on their stage of life. The outdoor access area must also be maintained as organic and records kept to document that it has been. This includes a history of how the fields have been used. Section 205.203 requires that all organic producers must take steps to prevent the contamination of water and minimize soil erosion. Soil and water tests should be done to monitor quality. For pastures this will also include the type and source of seeds used. It is also important to document at which age chicks are first given access to the outdoors.
§ 205.105: Other substances-
Cleaning/Disinfecting records
Only approved sanitizers are allowed when cleaning houses or facilities between flocks. Some sanitizers must be rinsed, while others do not require a rinse. Work with your certifier to determine sanitizers that are allowed. Document cleaning of houses and include date, sanitizer(s) used, and any other information required by your certifier.
As with any commercial operation, the number of eggs or birds produced should be recorded. This documentation is required for organic certification, but also gives the producer an idea of the level of production and early detection of any sudden drops. Daily feed consumption and water intake should also be monitored.
If non-organic products are being raised on the same farm, there must be documentation showing how the commingling of organic and non-organic products is prevented.
The type and amount of bedding material used (e.g., pine shavings) needs to be documented. If bedding materials are consumed by the birds, then the bedding materials must be organic, and records must be maintained to verify organic status.
Manure management is an important part of an integrated farming operation. Manure is a valuable byproduct of poultry production operations and a good source of nutrients for organic crop production. The amount of manure produced, and how it is used, needs to be documented.
Whether you are producing poultry meat or eggs, the products must be handled organically while being shipped for processing. For meat birds, this includes the certification of the facility where the birds are slaughtered, as well as the method and condition of transport to the slaughter facility. The slaughter facility must be certified organic, and its current certificate and documentation must be kept in your records. Similarly, if there is off-farm egg processing, the facility must be certified organic or covered under your certificate in order for the eggs to be labeled as such. If you are processing and packaging the eggs on-farm, the egg handling area and any materials used must be included in the overall organic plan for the farm.
Adapt your recordkeeping system to your own operationDifferent producers may need to keep different types of records to demonstrate compliance because of their activities or scale. While certain records are essential for most operations—such as feed purchase records—certifiers may require other records for some producers and not others if they feel that additional information is required to determine compliance with the regulations. In general, larger operations are required to keep more records than smaller operations. Again, your certifier will determine your compliance with National Organic Program standards. Be prepared to work with your certifier and adjust your recordkeeping system to meet the recordkeeping standards.
The records you are required to keep may also depend on how you market your eggs, live organic birds, or meat. If you sell on a wholesale basis to a single buyer, a summary of sales or transportation of birds may be adequate. Total income will also need to be reported. Certifiers rely on this information to ensure that non-organic product is not being sold as organic.
Producers marketing directly to consumers or to a variety of accounts are also required to document total sales. Sales information must include both the quantity of product sold and income from sales. Certifiers rely on this information to ensure that non-organic product is not being sold as organic.
You are required to make your records available during normal business hours to your certifier, state organic program (California only), and authorized representatives of the USDA. You are also required to keep your records on file for no less than five years.
How records might vary for large vesus small producers Large producers-
Outdoor Access Logs
Identify dates and reason(s) for confinement. Be specific. Most certifiers will require that you record animals' outdoor access on a daily basis. Additionally, a stated policy or standard operating procedure should describe any time periods when birds are typically confined, including the reason for such confinement (e.g. pullets until feathered). -
Facility records
- Diagram of each house
- Length and width of building
- Location and size of all doors
- Outdoor access areas, to scale
- Locations of feeders/waterers (indoors and outdoors)
- Temperature logs
- Cleaning/disinfection of houses between flocks
- Ammonia levels
-
Outdoor Access Logs
Document dates and reason(s) for confinement. Marking days on calendar, with the reason for confinement noted, will most likely be adequate to demonstrate compliance. -
Bird movement or location records (optional)
Small producers that move birds around pasture may wish to record the days when birds are moved. This can provide assurances to certifiers that the ground on which birds trod does not become contaminated by excess manure.
- United States Department of Agriculture. 2000. National organic program: Final rule. Codified at 7 C.F.R., part 205. (Available online at: http://www.ecfr.gov/cgi-bin/text-idx?tpl=/ecfrbrowse/Title07/7cfr205_mai...) (verified 13 April 2013)
This is an eOrganic article and was reviewed for compliance with National Organic Program regulations by members of the eOrganic community. Always check with your organic certification agency before adopting new practices or using new materials. For more information, refer to eOrganic's articles on organic certification.
eOrganic 7778
Twelve Steps Toward Ecological Weed Management in Organic Vegetables
eOrganic author:
Mark Schonbeck, Virginia Association for Biological Farming
Ecological weed management begins with careful planning of the cropping system to minimize weed problems, and seeks to utilize biological and ecological processes in the field and throughout the farm ecosystem to give crops the advantage over weeds. In addition, mechanical and other control measures are usually needed to protect organic crops from the adverse effects of weeds. This is particularly true in vegetables and other annual crops, for which production practices keep natural plant succession at its earliest stages, thereby eliciting the emergence of pioneer plants that can become agricultural weeds.
While tillage and cultivation can degrade soil quality and increase the risk of erosion losses, many other organic weed management tools (Table 1) are more soil-friendly. For example, a diversified rotation of vigorous cash crops and cover crops can enhance soil organic matter, tilth, and fertility, provided that a sufficient quantity and diversity of residues are returned to the soil to feed the soil life. Grazing livestock after a production crop to remove weeds or interdict weed seed set can add fertility in the form of manure, though intensive grazing can also compact the soil. In the interest of food safety, care must be taken to avoid direct contact of fresh manure with vegetables and other food crop. Mowing and flame weeding (if properly done to avoid excessive heating of the soil itself) are much easier on soil structure than cultivation, and can be just as effective in certain stages of weed and crop development. Mowing or rolling a cover crop to form an in situ mulch can enhance the soil benefits of the cover crop, compared to tilling it in, and can effectively suppress many annual weeds. Other organic mulches, such as straw and chipped brush, add organic matter, whereas synthetic clear or colored plastic films and weed barrier fabrics do not. All mulches are very effective in preventing soil erosion.
Table 1. A summary of organic weed management tools. Preventive Control Major tools: The Grower’s Mind (planning, observation, and ingenuity) X X Vigorous Cash Crops X Crop Rotation X Cover Crops X Organic Mulches X X Opaque Synthetic Mulches (black plastic, etc.) X X Conservation Biological Control (conserve weed consumers present on farm) X X Livestock X X Tillage and Cultivation Tools and Implements X Mowers and other Cutting Tools X Rollers and Roll-crimpers (for converting mature cover crops into in-situ mulch) X X Flame Weeders X Minor and experimental tools: OMRI certified organic herbicides X Bioherbicides (specific pathogens of weeds) X Management of soil microflora X X Specific crop–weed allelopathic interactions X X Classical biological controls for specific weeds (usually against invasive exotic weeds in rangeland and natural ecosystems) X Clear plastic mulch (soil solarization) X X
Ecological weed management consists of many-component strategies tailored to each region, cropping system, and farm. Matt Liebman and Eric Gallandt (1997) describe the process as using “many little hammers”, including “indirect controls”, such as crop variety, planting date, and nutrient management, rather than relying only on the “direct controls” or “large hammers” of cultivation and herbicides. In their words, “the use of a combination of methods can lead to (i) acceptable control through the additive, synergistic, or cumulative action of tactics that may not be effective when used alone, (ii) reduced risk of crop failure or serious loss by spreading the burden of protection across several methods, and (iii) minimal exposure to any one tactic, and consequently reduced rates at which pests adapt and become resistant.” (Liebman & Gallandt, 1997, p. 326)
The following list outlines twelve key steps toward successful organic weed management that are discussed in greater detail in a series of related articles that can be found on this website. Note that these steps do not comprise a precise linear sequence of instructions; rather they offer a conceptual framework within which each farmer can develop a site-specific strategy. This process requires systems thinking, which views the field as a complex system of interacting components—such ascrops, weeds, soil, insects, and microorganisms—that form a web of relationships, not a linear sequence of cause-and-effect. Similarly, the following steps are employed together in a synergistic manner, and thus differ from the sequence of instructions for assembling a car or a farm implement. For example, Step 6 (cover crops) can be seen as a part of Step 2 (minimize niches for weeds), and Step 1 (know the weeds) provides vital information for other steps, particularly steps 3 (keep the weeds guessing), 4 (design for effective weed control), and 7 (manage the weed seed bank). Biological processes (Step 9) include indigenous biocontrols that help reduce the weed seed bank (Step 7) as well as the competitive and allelopathic effects of cover crops (Step 6). Step 11 (observe weeds and adapt practices) is an ongoing feedback loop that informs and fine-tunes all the other steps. Utilizing this or another suitable framework, the organic grower selects and assembles a set of “many little hammers” that, working together, keep the farm’s weeds from becoming major weed problems.
Planning Steps1. Know the Weeds Obtain correct identification of the major weeds present on the farm. Monitor fields regularly throughout the season. Keep records on what weeds emerge at different seasons, and on efficacy of any preventive and control measures taken. Learn each weed’s life cycle, growth habit, seasonal pattern of development and flowering, modes of reproduction and dispersal, seed dormancy and germination, and how the weed affects crop production. Find the weed’s weak points—possibly the stages in its life cycle that are most vulnerable to control tactics—and stresses to which the weed is sensitive; these can be exploited in designing a management strategy.
"Know the weeds" is listed first because it informs most of the succeeding steps. However, gaining a thorough knowledge of the farm’s weed flora is an ongoing process over many seasons (perhaps the lifetime of the farmer!) that drives the year-to-year refinement of the farm’s weed management system.
2. Design the Cropping System to Minimize Niches for Weed Growth In planning the crop rotation, avoid creating open niches in time or space. Plan tight rotations that follow one crop harvest promptly with the next planting. Open niches in space between crop rows can be reduced by using a narrower row spacing, intercropping, relay cropping, overseeding cover crops into established vegetables, or no-till management of cover crops prior to transplanting vegetables.
3. Keep the Weeds Guessing with Crop Rotations Plan and implement diversified crop rotations that vary timing, depth, frequency, and methods of tillage; timing and methods of planting, cultivation, and harvest; as well as crop plant family. Alternate warm- and cool-season vegetables. Rotate vegetable fields into perennial cover for two or three years to interrupt life cycles of annual weeds adapted to frequent tillage. Schedule tillage and cultivation operations when they will do the most damage to the major weed species.
4. Design the Cropping System and Select Tools for Effective Weed Control Develop control strategies to address anticipated weed pressures in each of the farm’s major crops. Choose the best cultivation implements and other tools for cost-effective preplant, between-row, and within-row weed removal. Plan bed layout, as well as row- and plant spacing, to facilitate precision cultivation. Choose irrigation methods and other cultural practices that are compatible with planned weed control operations.
Preventive Steps During the Season5. Grow Vigorous, Weed-competitive Crops A healthy, fast-growing crop that can outcompete weeds is the best way to prevent weed problems. Choose locally-adapted crop varieties that grow tall or form lots of foliage that can shade out weeds. Maintain healthy, living soil. Provide optimum growing conditions—planting date and spacing, moisture, soil tilth and aeration, fertility, and pest and disease management. Deliver water and fertilizer within-row to feed the crop and not the weeds. Note that either insufficient or excessive levels of major nutrients (nitrogen, phosphorus, and potassium) can give certain weeds a competitive advantage over the crops.
6. Put the Weeds Out of Work—Grow Cover Crops! Cover crops do the same job as weeds, only better. They rapidly occupy open niches, protect and restore the soil, provide beneficial habitat, add organic matter, and hold and recycle soil nutrients. They suppress weeds through direct competition and sometimes through allelopathy—the release of plant-growth-inhibiting substances into the soil. Whenever a bed or field becomes vacant, plant a cover crop immediately so that it can begin the vital restorative work that nature accomplishes with pioneer plants or weeds. Good cover cropping plays a major role in Step 2 (minimzing open niches), and can put the weeds out of a job.
7. Manage the Weed Seed Bank—Minimize “Deposits” and Maximize “Withdrawals” Prevent formation and release of viable weed seeds, and proliferation of rhizomes and other propagules of perennial weeds. Avoid importing new weeds with manure, mulch hay, and other materials from off-farm sources. Utilize stale seedbed, cultivated fallow, or targeted tillage practices to draw down seed banks of the major weeds present. Encourage weed seed mortality and weed seed consumption by ground beetles and other organisms (see Step 9 below).
Control Steps During the Season8. Knock Out Weeds at Critical Times Plant vegetables into a clean seedbed, hit early-season weeds while the are small, and keep crops clean through their critical weed free period (through the first third or half of the life cycle of most vegetables). Prevent seed set by “escapes” and late season weeds. When practical, interrupt vegetative propagation by invasive perennial weeds through timely removal of top growth.
9. Utilize Biological Control Processes to Further Reduce Weed Pressure Rotate livestock, poultry, or weeder geese through fields to graze weeds and interrupt seed set. To ensure food safety and comply with USDA Organic Standards, time such grazing so that fresh droppings are not deposited any less than 120 days prior to harvest of the next crop. Encourage weed seed predation and decay by maintaining high soil biological activity and providing habitat (mulch, cover crops, hedgerows) for belowground and aboveground weed seed consumers (conservation biological control). Enhance overall soil biological activity to tip the competitive balance in favor of crops, and possibly to shorten the "half life" of the weed seed bank.
Classical biological controls (introduced natural enemies) are commercially available for a few invasive exotic weeds.
10. Bring Existing Weeds Under Control Before Planting Weed-sensitive Crops Weed control in perennial horticultural crops like asparagus, small fruit, and some cut flowers can be quite difficult, especially when perennial weeds dominate the weed flora. Bring existing weed pressures under good control through repeated tillage and intensive cover cropping before planting any perennial vegetable, fruit, or ornamental crops. Choose fields with the best weed control or lowest weed pressure for weed-sensitive annual vegetables with a long critical weed free period, such as carrot, onion ,and parsnip. Be sure weeds, especially perennial weeds, are under good control before attempting no-till management of cover crops prior to cash crop planting.
Enhancing the Organic Weed Management System – Observe, Adapt, Experiment11. Keep Observing the Weeds and Adapt Practices Accordingly Note and record any changes in weed species composition, emergence and growth pattern, or weed pressure, and modify practices as needed. For example, an increase in certain annual “weeds of cultivation” may indicate a need to reduce tillage or diversify the crop rotation. An increase in invasive perennials may require tilling deeper or more aggressively for a time. Watch out for the arrival of new weed species that could pose problems.
Expect weed populations and flora to shift over time. Every farm decision and field operation can elicit changes in the weed community, as can weather variations, to say nothing of long term climate changes. “Reading” the weeds each year becomes an information feedback loop, guiding weed management practices for the following season.
12. Experiment Try out new tactics and strategies to deal with major weed challenges. Farmers continually develop innovative strategies based on new tools that they fashion themselves or that researchers develop, new uses for old tools, and new combinations of preventive and control tactics. In the words of University of Vermont Extension Specialist Vern Grubinger (1997):
Experiment to fine-tune your weed management tactics.
- Start on a small scale with tools and techniques that are new to your farm.
- Identify your most problematic weeds and compare different combinations or rotations, cover crops, and cultivation tools to see how effective they are in providing control.
- Keep an eye out for new tools, or new ways to use old tools.
- Leave a control row or section untreated, so you can see the effectiveness of your tactics.
~ Grubinger, 1997.
Part of experimenting is to watch for new developments. Researchers and farmers continue to explore and expand the horizons of possibility in ecological and organic weed management. Check farming magazines and publications for practical applications of their work, from new cultivation tools to new strategies for particularly stubborn weed problems. Some cutting edge areas of research may take longer to yield practical results, yet bear watching and possible integration into a farm’s weed management strategy. These range from natural herbicides, bioherbicides, and classical biological controls, to specific weed–crop allelopathic interactions and manipulation of weed–crop–soil–microbe relationships to give the crop a competitive edge over certain weeds.
While none of these endeavors is likely to yield a “big hammer” to replace herbicides or steel, they can contribute additional “little hammers” to enhance efficacy and reduce the amount of tillage and cultivation needed.
Caution: This is Not a CookbookAn effective organic weed management system cannot be spelled out precisely because ecological weed management is inherently site specific and responsive to changes in the farm ecosystem. There is effectively no “organic weed control cookbook” to replace the precise herbicide protocols that have been developed for conventional production of row crops and some vegetables. No scientist can come up with a better weed management strategy for a particular farm than the strategy a skillful organic farmer can develop by applying ecological weed management principles to the particular suite of crops, weeds, soil conditions, and available resources on her or his farm.
This outline is not the only valid roadmap available. There is nothing set-in-stone about the number of steps or the order in which they appear here. Other outlines for ecological weed management have been developed for particular regions and cropping systems; these may be more directly applicable to your farm or situation. See the References and Additional Resources below for some specific examples.
References and Citations
- Grubinger, V. 1997. 10 steps toward organic weed control. American Vegetable Grower 46: 22–24.
- Liebman, M., and E. R. Gallandt. 1997. Many little hammers: ecological approaches for management of crop–weed interactions. p. 291–343. In L. E. Jackson (ed.) Ecology in agriculture. Academic Press, San Diego, CA.
- Finney, D. M., and N. G. Creamer. 2008. Weed management on organic farms. Center for Environmental Farming Systems organic production guide [Online]. North Carolina Cooperative Extension Service, Raleigh, NC. Available at: http://www.cefs.ncsu.edu/resources/organicproductionguide/weedmgmtjan808accessible.pdf (verified 11 March 2010).
- Mohler, C. Undated. Ecology of weed management in organic systems [Online slide presentation]. Available at: http://www.css.cornell.edu/WeedEco/Mohler Ecology of Weed manag Presentation.pdf (verified 11 March 2010).
- Sullivan, P. 2003. Principles of sustainable weed management for croplands [Online]. ATTRA Publication #PO39. National Sustainable Agriculture Information Service. Available at: https://attra.ncat.org/attra-pub-summaries/?pub=109 (verified 4 June 2019).
This is an eOrganic article and was reviewed for compliance with National Organic Program regulations by members of the eOrganic community. Always check with your organic certification agency before adopting new practices or using new materials. For more information, refer to eOrganic's articles on organic certification.
eOrganic 2320
Soil Health and Organic Farming Webinar Series
The Organic Farming Research Foundation and eOrganic presented a series of 9 webinars focused on soil health topics: building organic matter, weed management, conservation tillage, cover crops, plant breeding and variety selection, water management and quality, nutrient management, and more! This series is recommended for farmers, extension agents, educators, agricultural professionals, and others interested in building soil health. Presenters Mark Schonbeck and Diana Jerkins of the Organic Farming Research Foundation reviewed the most recent research on soil health practices and explored how organic growers can build healthy soils on their operations. The webinars provide practical guidelines for growers and in-depth analysis of research outcomes. Scroll down to view the recordings, slides and accompanying presentation notes. The Organic Farming Research Foundation has also produced a series of Soil Health Guides which cover the topics in these webinars. Download the guides at https://ofrf.org/soil-health-and-organic-farming-ecological-approach.
May 9, 2018: Building Organic Matter for Healthy Soils: An OverviewWe will discuss the attributes of healthy soil, the central role of organic matter, and how to monitor and enhance soil health in organic production. The presentation will outline key organic practices for building soil organic matter and optimizing soil functions in relation to fertility, crop yield, and resource conservation.
'
June 13, 2018: Weed Management: An Ecological ApproachThis webinar will focus on integrated organic weed management tools and practices that give crops the edge over weeds, build soil health, and reduce the need for soil disturbance. Slide handout
September 19, 2018: Practical Conservation TillageThis webinar includes the impacts of tillage on soil health, including practical, soil-friendly tillage practices for organic systems. We will discuss several newer tillage tools and approaches that reduce adverse impacts on soil life and soil structure.
Slide handout, Presentation notes
October 17, 2018: Cover Crops: Selection and ManagementThis webinar will focus on selecting the best cover crops, mixes, and management methods for soil health, including crop rotations and cropping system biodiversity.
Slide handout, Presentation notes
November 14, 2018: Plant Genetics: Plant Breeding and Variety SelectionThis webinar will cover plant breeding and variety selection for performance in sustainable organic systems, including nutrient and moisture use efficiency, competitiveness toward weeds, and enhanced interactions with beneficial soil biota. We will also discuss heritable traits that could directly benefit soil biology and soil health.
Slide handout Presentation notes
January 9, 2019: Water Management and Water QualityThis webinar will focus on the role of soil health and organic soil management in water conservation and water quality.
Slide handout, Presentation notes
February 20, 2019: Nutrient Management for Crops, Soil and the EnvironmentThis webinar includes a discussion of the role of soil health and the soil food web, including practical guidelines for optimizing crop nutrition, minimizing adverse environmental impacts of organic fertility inputs, and adapting soil test-based nutrient recommendations (especially N) for organic systems.
Slide handout, Presentation notes
March 20, 2019: Organic Practices for Climate Mitigation, Adaptation, and Carbon SequestrationIn this webinar, we will discuss the capacity of sustainable organic systems and practices to sequester soil carbon, minimize nitrous oxide and methane emissions during crop and livestock production, and enhance agricultural resilience to weather extremes. The presentation will include practical guidelines for optimizing the organic farm’s “carbon footprint” and adaptability to climate disruptions already underway.
Slide handout, Presentation notes
May 22, 2019: Understanding and Managing Soil Biology for Soil Health and Crop ProductionThis webinar will examine the functions of the soil food web and key components thereof in promoting soil health and fertility and sustainable organic crop production. Research-based guidance on organic practices and NOP-approved inputs for improved soil food web function will be discussed.
Slide handout, Presentation notes
About the PresentersMark Schonbeck is a Research Associate at the Organic Farming Research Foundation. He has worked for 31 years as a researcher, consultant, and educator in sustainable and organic agriculture. He has participated in on-farm research into mulching, cover crops, minimum tillage, and nutrient management for organic vegetables. For many years, he has written for the Virginia Association for Biological Farming newsletter and served as their policy liason to the National Sustainable Agriculture Coalition. He has also participated in different research projects to analyze, evaluate and improve federally funded organic and sustainable agriculture programs. In addition, Mark offers individual consulting in soil test interpretation, soil quality and nutrient management, crop rotation, cover cropping, and weed management.
Diana Jerkins is the Research Program Director of the Organic Farming Research Foundation. She has decades of experience in agricultural research, federal program management, university administration and hands-on farming. She was a National Program Leader with the US Department of Agriculture’s National Institute of Food and Agriculture (NIFA) between 2002 and 2014, and she helped implement the agency’s first sustainable and organic agriculture programs.
Thank you to the Clarence E. Heller Charitable Foundation for supporting this project.
This is an eOrganic article and was reviewed for compliance with National Organic Program regulations by members of the eOrganic community. Always check with your organic certification agency before adopting new practices or using new materials. For more information, refer to eOrganic's articles on organic certification.
eOrganic 25148